Accurate Multi-Element Analysis of Whole Blood
App Note / Case Study
Published: March 19, 2025
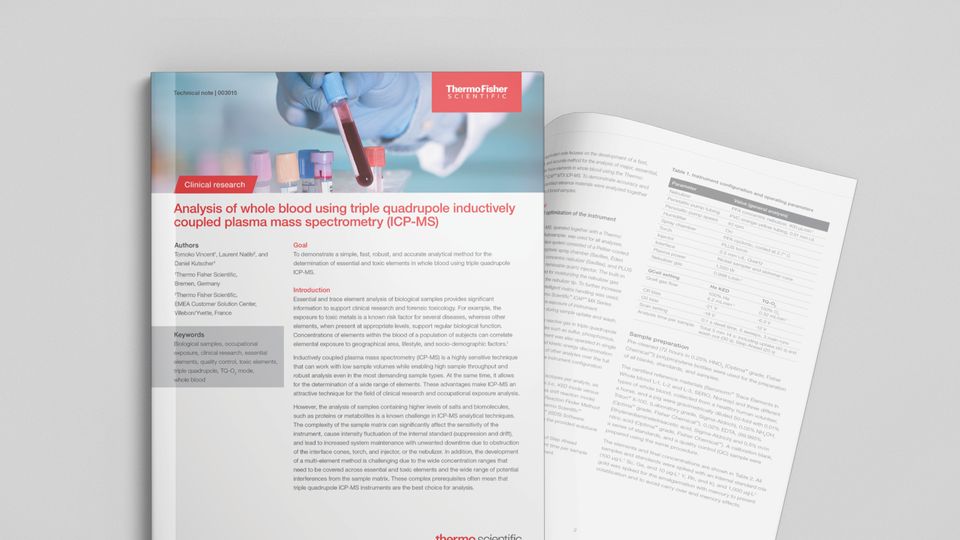
Credit: Thermo Fisher Scientific
Essential and toxic element analysis in biological samples is critical for clinical research and occupational exposure assessment. However, complex sample matrices can introduce spectral interferences, affecting accuracy and sensitivity.
A robust method using triple quadrupole ICP-MS enables high-throughput, interference-free analysis of major, essential and trace elements in whole blood.
This application note highlights a validated approach that ensures precise, reliable results across a broad concentration range.
Download this application note to explore:
- A high-sensitivity method for analyzing 43 elements in whole blood
- Strategies to eliminate interferences using oxygen
- Optimized workflows for improved productivity and minimal instrument downtime
Goal
To demonstrate a simple, fast, robust, and accurate analytical method for the
determination of essential and toxic elements in whole blood using triple quadrupole
ICP-MS.
Introduction
Essential and trace element analysis of biological samples provides significant
information to support clinical research and forensic toxicology. For example, the
exposure to toxic metals is a known risk factor for several diseases, whereas other
elements, when present at appropriate levels, support regular biological function.
Concentrations of elements within the blood of a population of subjects can correlate
elemental exposure to geographical area, lifestyle, and socio-demographic factors.1
Inductively coupled plasma mass spectrometry (ICP-MS) is a highly sensitive technique
that can work with low sample volumes while enabling high sample throughput and
robust analysis even in the most demanding sample types. At the same time, it allows
for the determination of a wide range of elements. These advantages make ICP-MS an
attractive technique for the field of clinical research and occupational exposure analysis.
However, the analysis of samples containing higher levels of salts and biomolecules,
such as proteins or metabolites is a known challenge in ICP-MS analytical techniques.
The complexity of the sample matrix can significantly affect the sensitivity of the
instrument, cause intensity fluctuation of the internal standard (suppression and drift),
and lead to increased system maintenance with unwanted downtime due to obstruction
of the interface cones, torch, and injector, or the nebulizer. In addition, the development
of a multi-element method is challenging due to the wide concentration ranges that
need to be covered across essential and toxic elements and the wide range of potential
interferences from the sample matrix. These complex prerequisites often mean that
triple quadrupole ICP-MS instruments are the best choice for analysis.
Analysis of whole blood using triple quadrupole inductively
coupled plasma mass spectrometry (ICP-MS)
Technical note | 003015
Authors
Tomoko Vincent1
, Laurent Naëls2
, and
Daniel Kutscher1
1
Thermo Fisher Scientific,
Bremen, Germany
2
Thermo Fisher Scientific,
EMEA Customer Solution Center,
Villebon/Yvette, France
Keywords
Biological samples, occupational
exposure, clinical research, essential
elements, quality control, toxic elements,
triple quadrupole, TQ-O2
mode,
whole blood
Clinical research
This application note focuses on the development of a fast,
robust, and accurate method for the analysis of major, essential,
and other trace elements in whole blood using the Thermo
Scientific™ iCAP™ MTX ICP-MS. To demonstrate accuracy and
precision, certified reference materials were analyzed together
with a range of blood samples.
Experimental
Experimental optimization of the instrument
parameters
An iCAP MTX ICP-MS, operated together with a Thermo
Scientific™ iSC-65 Autosampler, was used for all analyses.
The sample introduction system consisted of a Peltier-cooled
(2.7 °C), baffled PFA cyclonic spray chamber (Savillex, Eden
Prairie, MN, USA), PFA concentric nebulizer (Savillex), and PLUS
torch with a 2.5 mm i.d. removable quartz injector. The built-in
argon humidifier was used for moisturizing the nebulizer gas
to prevent salt deposit on the nebulizer tip. To further increase
uptime of the instrument, intelligent matrix handling was used,
a unique feature of the Thermo Scientific™ iCAP™ MX Series
ICP-MS instruments to reduce exposure of instrument
components to sample matrix during sample uptake and wash.
In addition to the use of O2
as a reactive gas in triple quadrupole
mode for highly interfered analytes such as sulfur, phosphorous,
arsenic, and selenium, the instrument was also operated in single
quadrupole mode using helium and kinetic energy discrimination
(KED) for interference-free analysis of other analytes over the full
mass range. Table 1 summarizes the instrument configuration
and analytical parameters.
The selection of the most appropriate isotopes per analyte, as
well as the optimum analysis conditions (i.e., KED mode versus
reactive gas, on mass mode versus mass shift reaction mode)
was automatically recommended by the Reaction Finder Method
Development Assistant available in the Thermo Scientific™
Qtegra™ Intelligent Scientific Data Solution™ (ISDS) Software.
Measurement modes were optimized using the provided autotune
procedures.
The iSC-65 autosampler allowed for the use of Step Ahead
functionality to reduce the overall measurement time per sample
and increase the productive time of the instrument.
Sample preparation
Pre-cleaned (72 hours in 0.25% HNO3
(Optima™ grade, Fisher
Chemical™)) polypropylene bottles were used for the preparation
of all blanks, standards, and samples.
The certified reference materials (Seronorm™ Trace Elements in
Whole blood L-1, L-2 and L-3, SERO, Norway) and three different
types of whole blood, collected from a healthy human volunteer,
a horse, and a pig were gravimetrically diluted 50-fold with 0.01%
Triton™ X-100, (Laboratory grade, Sigma-Aldrich), 0.05% NH4OH,
(Optima™ grade, Fisher Chemical™), 0.02% EDTA, (99.995%
Ethylenediaminetetraacetic acid, Sigma-Aldrich) and 0.5% m/m
nitric acid (Optima™ grade, Fisher Chemical™). A calibration blank,
a series of standards, and a quality control (QC) sample were
prepared using the same procedure.
The elements and final concentrations are shown in Table 2. All
samples and standards were spiked with an internal standard mix
(100 μg·L-1 Sc, Ge, and 10 μg·L-1 Y, Rh, and Ir), and 1,000 µg·L-1
gold was spiked for the amalgamation with mercury to prevent
volatilization and to avoid carry over and memory effects.
Parameter Value (general analysis)
Nebulizer PFA concentric nebulizer, 400 μL·min-1
Peristaltic pump tubing PVC orange-yellow tubing, 0.51 mm i.d.
Peristaltic pump speed 40 rpm
Humidifier On
Spray chamber PFA cyclonic, cooled at 2.7° C
Torch PLUS torch
Injector 2.5 mm i.d., Quartz
Interface Nickel sampler and skimmer cone
Plasma power 1,550 W
Nebulizer gas 0.998 L·min-1
QCell setting He KED TQ-O2
Qcell gas flow 100% He
4.2 mL·min-1
100% O2
0.32 mL·min-1
CR bias -21 V -6.3 V
Q3 bias -18 V -12 V
Scan setting 0.1 s dwell time, 5 sweeps, 3 main runs
Analysis time per sample Total 3 min 14 s: including uptake (40 s) and
wash out (30 s), Step Ahead (20 s)
Table 1. Instrument configuration and operating parameters
2
Results and discussion
Sensitivity and linearity
Table 3 summarizes the analysis conditions, instrument detection
limits (IDLs), method detection limits (MDL) obtained, together with
the coefficient of determination (R2
) for all 43 elements analyzed in
this study. The correlation coefficients (R2
) obtained for all analytes
were found to be greater than 0.9995, which suggests excellent
linear response for the established concentration range for each
analyte. The IDLs were calculated as three times the standard
deviation of ten replicate measurements of the calibration blank.
MDLs for all 43 elements measured are based on the instrumental
detection limits summarized above but include the dilution factor
50, incurred during the sample preparation process.
Table 2. Summary of the concentration details of the standard calibration, CCV (continuing calibration verification. All numbers are shown
in µg·L-1.
Standard group STD-1 STD-2 STD-3 STD-4 STD-5 STD-6 STD-7 QC (CCV)
Al, As, Ag, Ba, Bi, Co, Cr, Ni, V, U, Pb, Mn,
Ga, Tl, Se, Cd, In
0.002 0.025 0.05 0.2 1 10 50 0.2
Na, Mg, Fe, Si, P, K, Ca, S 200 1,000 10,000 50,000 - - - 1,000
Mo, Nb, Re, Ta, Ti, Ze, W 0.02 0.1 1 5 10 - - 0.1
Sn, Sb, Pt 0.01 0.05 0.1 - - - - 0.05
Hg, B, Li, Be 0.1 0.5 1 - - - - 0.5
Cu, Zn, Rb, Sr 0.05 0.2 1 10 50 200 - 10
Mode Q3 analyte Q1 Resolution Q3 Resolution Internal standard R2 LOD MDL
7
Li TQ-O2
7
Li High Normal Sc >0.9999 0.009 0.43
9Be TQ-O2
9Be High Normal Sc >0.9999 0.014 0.71
11B TQ-O2
11B High Normal Sc 0.9999 0.068 3.41
23Na He KED - - Normal Sc >0.9999 0.635 31.8
24Mg He KED - - Normal Sc >0.9999 0.049 2.43
27Al TQ-O2
27Al iMS Normal Sc 0.9999 0.016 0.81
28Si TQ-O2
28Si.16O High High Sc >0.9999 0.173 8.65
31P TQ-O2
31P.16O High High Sc 0.9999 0.270 13.5
33S TQ-O2
33S.16O High High Sc 0.9999 7.223 361.2
39K TQ-O2
39K iMS Normal Sc >0.9999 0.102 5.10
45Sc He KED - - Normal Internal standard
45Sc TQ-O2
15Sc.16O High High Internal standard
44Ca He KED - - Normal Sc 0.9999 1.6 79.6
49Ti TQ-O2
49Ti.16O High Normal Sc 0.9998 0.008 0.52
51V TQ-O2
51V.16O High Normal Sc >0.9999 0.001 0.04
52Cr TQ-O2
52Cr.16O High Normal Sc 0.9998 0.013 0.66
55Mn TQ-O2
55Mn High Normal Sc 0.9995 0.004 0.20
57Fe He KED - - Normal Sc >0.9999 2.4 118.0
59Co TQ-O2
59Co High Normal Ge >0.9999 0.001 0.03
60Ni He KED - - Normal Ge >0.9999 0.006 0.32
63Cu TQ-O2
63Cu iMS Normal Ge >0.9999 0.009 0.47
66Zn TQ-O2
66Zn iMS Normal Ge 0.9999 0.035 1.76
71Ga He KED - - Normal Ge >0.9999 0.005 0.24
72Ge He KED - - Normal Internal standard
72Ge TQ-O2
72Ge iMS Normal Internal standard
75As TQ-O2
75As.16O High Normal Ge 0.9994 0.010 0.48
80Se TQ-O2
80Se.16O iMS Normal Ge >0.9999 0.010 0.50
Table 3 (part 1). Summary of analysis mode, and calibration results, R2, LODs, and MDLs for all analytes. All numbers are shown
in µg·L-1.
3
Accuracy and polyatomic interference removal for the
analysis of whole blood
The process of interference removal using oxygen is shown in
Figure 1 using arsenic and titanium as examples. The analyte
of interest reacts with oxygen and forms a molecular ion with a
new mass-to-charge ratio (referred to as a mass-shift reaction),
and the previously isobaric (i.e., having the same nominal mass)
interference does not react in a similar way and can therefore
be eliminated using the third quadrupole of the system. The first
quadrupole provides an additional mass filtration before the
collision/reaction cell, so that unwanted side reactions with other
components present in the ion beam are effectively suppressed.
One element often analyzed in patients’ samples is titanium,
which is monitored to track potential degradation of titaniumbased orthopedic and dental implants. Following recent research
on the possible carcinogenic effects of titanium dioxide, the fate
of titanium in the human body has become a growing area of
clinical research focus. Due to the high concentrations of calcium
in biological samples, the main isotope of titanium (48Ti, 73.7%
abundant in nature) has an isobaric interference with 48Ca. To
detect titanium at relevant levels, often a mass shift reaction
using NH3
is applied, which selectively forms 48TiNH(NH3)
3,
detectable at m/z 114, whereas 48Ca does not react. The use of
NH3, however, is not always possible due to safety restrictions
within the laboratory. In this study, a less abundant isotope of
titanium was monitored (49Ti, 5.41% abundant in nature) using
TQ-O2
mode. This allowed comparable detection limits to be
achieved.3
Mode Q3 analyte Q1 Resolution Q3 Resolution Internal standard R2 LOD MDL
85Rb TQ-O2
85Rb iMS Normal Y 0.9997 0.001 0.03
88Sr He KED - - Normal Y >0.9999 0.003 0.14
89Y He KED - - Normal Internal standard
89Y TQ-O2
89Y.16O iMS Normal Internal standard
90Zr TQ-O2
90Zr.16O iMS Normal Y 0.9999 0.002 0.12
93Nb He KED - - Normal Y 0.9998 0.000 0.02
95Mo He KED - - Normal Y 0.9999 0.008 0.42
103Rh He KED - - Normal Internal standard
103Rh TQ-O2
103Rh iMS Normal Internal standard
107Ag TQ-O2
107Ag iMS Normal Rh >0.9999 0.002 0.12
111Cd TQ-O2
111Cd iMS Normal Rh >0.9999 0.009 0.43
115In He KED - - Normal Rh 0.9999 0.001 0.06
115In TQ-O2
115In iMS Normal Rh >0.9999 0.001 0.05
118Sn TQ-O2
118Sn iMS Normal Rh >0.9999 0.001 0.07
121Sb TQ-O2
121Sb iMS Normal Rh 0.9988 0.004 0.20
137Ba He KED - - Normal Rh >0.9999 0.008 0.40
181Ta He KED - - Normal Ir 0.9996 0.000 0.01
182W He KED - - Normal Ir 0.9999 0.000 0.02
185Re He KED - - Normal Ir 0.9998 0.000 0.02
193Ir He KED - - Normal Internal standard
193Ir TQ-O2
193Ir iMS Normal Internal standard
195Pt TQ-O2
195Pt iMS Normal Ir 0.9998 0.002 0.12
202Hg He KED - - Normal Ir 0.9999 0.002 0.12
205Tl He KED - - Normal Ir 0.9997 0.001 0.04
208Pb He KED - - Normal Ir >0.9999 0.001 0.05
209Bi He KED - - Normal Ir >0.9999 0.001 0.03
238U He KED - - Normal Ir >0.9999 0.000 0.02
Table 3 (part 2). Summary of analysis mode, and calibration results, R2, LODs, and MDLs for all analytes. All numbers are shown
in µg·L-1.
4
Achieving high sensitivity is important especially when analyzing
toxic elements in the diluted whole blood samples, in which
these elements are often present in ultra-trace amounts. The
use of collision cell technology is often associated with a loss of
sensitivity in the low mass range, which is often the reason for
measuring analytes such as lithium or beryllium using “No gas” or
“Standard” mode. However, as opposed to the use of KED mode,
reactive modes generally apply a negative bias potential at the
outlet of the collision/reaction cell (CRC), so that some of the ion
losses caused by collisions with gas molecules can be overcome.
The absolute sensitivity is typically not negatively affected, or
even may be significantly enhanced when using TQ-O2
mode, so
that low mass analytes detection limits in the sub ng·L-1 range can
be achieved (Figure 2).
The accuracy and precision of the analytical method was
assessed by analyzing commercially available certified reference
materials (CRMs) with different elemental concentration levels.
The exact concentrations in each level are summarized in
Table 4. The results obtained show that the calculated
concentrations of the target elements matched the certified
values, demonstrating the accuracy of method. For all reference
materials, nine individual samples were measured so that the
method was also demonstrated to deliver excellent precision
(Table 4). In addition to the CRMs, three different blood samples
were analyzed as technical replicates to assess the repeatability
of the results over longer analysis times.
Figure 1. Schematic showing the use of TQ-O2
mode and a mass shift reaction for interference free detection of
arsenic (As) and titanium (Ti)
Figure 2. Sensitivity comparison between He KED (normalized as 1) and TQ-O2
mode for selected analytes
0
2
4
6
8
Ratio
He KED TQ-O2
LI B Ti As Se Cd
5
Table 4. Quantitative results obtained for the CRM whole blood samples. Analyte concentrations are reported as µg·L-1. All numbers annotated
with * are known reference values (expected values).
MDL CRM L1
values
Measured L1
(n=9)
L1 RSD
(%)
CRM L2
values
Measured L2
(n=9)
L2 RSD
(%)
CRM L3
values
Measured L3
(n=9)
L3 RSD
(%)
7
Li 0.43 0.37 0.45 14.3 11.00 11.49 4.0 0.73* 0.57 8.0
9Be 0.71 <0.02 <MDL N.D. 5.50 5.49 15.1 10.10 10.84 10.6
11B 3.41 - 87.4 1.2 - 44.1 4.4 - 321.1 1.1
23Na 31.8 1,598,000* 1,609,898 1.1 1,618,000* 1,582,356 0.7 1,512,000* 1,545,066 1.0
24Mg 2.43 152,000 16,210 0.7 41,000 41,838 0.3 136,000* 14,737 0.5
27Al 0.81 10.40 13.76 1.8 57.00 52.72 0.4 88.00 89.53 1.3
28Si 8.65 - 1701 2.0 - 1,841 4.3 - 2,626 2.8
31P 13.5 203,000* 201,616 0.7 200,000* 194,496 0.2 187,000* 192,525 1.2
33S 361.2 957,000* 990,933 0.8 984,000* 978,749 0.4 921,000* 916,908 1.4
39K 5.10 1,089,000* 1,153,957 1.4 1,078,000* 1,121,154 1.0 1,037,000* 1,098,131 2.0
44Ca 79.6 158,000 17,205 1.2 56,000 58,192 0.6 141,000* 16182 0.6
49Ti 0.52 - 5.34 6.9 - 3.33 4.7 - 14.96 3.8
51V 0.04 0.26 0.26 1.5 3.10 3.34 1.1 4.40 4.53 1.7
52Cr 0.66 0.77 0.81 4.9 10.00 9.71 4.9 35.50 34.65 1.2
55Mn 0.20 19.70 21.10 0.8 24.20 21.62 0.3 33.30 31.49 1.4
57Fe 118.0 334,000* 327,397 0.7 335,000* 326,225 0.6 300,000* 288,596 0.8
59Co 0.03 0.22 0.31 3.8 5.00 4.87 0.3 10.30 10.22 0.6
60Ni 0.32 2.13 3.58 2.5 9.20 8.50 2.0 11.00 11.48 1.0
63Cu 0.47 640 601 0.3 940 842 0.2 2,080 1,938 0.1
66Zn 1.76 4,600 4,307 0.3 5,800 5,202 0.3 8,360 7,519 0.2
71Ga 0.24 0.05* <MDL N.D. 0.04* <MDL N.D. 0.04* <MDL N.D.
75As 0.48 2.1 2.2 0.9 12.2 12.2 0.8 27.3 29.0 0.8
80Se 0.50 69 67 0.1 144 126 0.1 198 223 0.0
85Rb 0.03 1,420* 1,443 0.7 1,460* 1,406 0.3 1180* 1275 0.4
88Sr 0.14 41.00 39.66 4.7 75.00 68.82 28.4 37.00 39.13 5.0
90Zr 0.12 0.41* 0.34 15.2 0.28* 0.17 13.6 0.28* 0.33 12.8
93Nb 0.02 0.03* 0.01 8.4 0.03* 0.02 2.4 0.04* 0.04 3.1
95Mo 0.42 0.37 0.38 29.6 4.50 4.46 0.9 6.90 6.59 1.6
107Ag 0.12 0.10 0.42 5.7 9.70 10.26 1.4 0.08 0.50 1.5
111Cd 0.43 0.28 0.26 16.0 5.10 4.63 3.8 9.90 9.81 1.6
115In 0.05 - <MDL 2.0 - 0.03 0.4 - 0.07 0.6
118Sn 0.07 0.2 0.2 3.0 4.7 4.0 0.6 9.9 9.5 0.3
121Sb 0.20 3.3 2.8 0.3 22.3 18.9 1.0 21.9 18.4 0.3
137Ba 0.40 427* 458 0.8 145* 153 1.6 557* 581 1.1
181Ta 0.01 <0.001* <MDL N.D. <0.001* <MDL N.D. <0.001 <MDL N.D.
182W 0.02 - <MDL N.D. - <MDL N.D. - <MDL N.D.
185Re 0.02 0.001* <MDL N.D. 0.0015 <MDL N.D. <0.001 <MDL N.D.
195Pt 0.12 0.004* <MDL N.D. 0.003* <MDL N.D. 0.01 <MDL N.D.
202Hg 0.12 1.57 1.57 1.8 16.60 14.79 1.3 25.80 22.0 1.4
205Tl 0.04 0.01 <MDL 0.5 10.10 8.04 0.4 25.20 21.57 0.4
208Pb 0.05 10.00 8.99 6.0 303 273 0.9 389 321 0.4
209Bi 0.03 0.01 <MDL 1.8 4.90 4.69 1.8 47.00 41.54 2.2
238U 0.02 0.13* 0.18 4.5 0.20* 0.25 5.2 0.12* 0.22 8.4
6
Some of the essential elements are found in high concentrations,
however other commonly present metals such as vanadium,
chromium, cobalt, arsenic, and selenium are typically found
only in concentrations below 10 µg·L-1 in whole blood. However,
there are many complex spectral interferences from the matrix,
formed from elements such as sodium, magnesium, phosphorus,
sulfur, potassium, calcium, and iron. These major elements
generate different types of interferences, including oxides,
isobaric interferences, or peak tailing for the target analyte. Thus,
complete removal of these interferences is a key prerequisite to
a successful method and was accomplished by using the TQ-O2
mode, as demonstrated by the results.
Table 5 (next page) shows the results obtained for the three
different whole blood samples and provides detailed information
on the concentrations for both toxic and essential elements.
Some analytes showed significant variation in the concentrations
of toxic or essential elements between them. The capability of
ICP-MS to provide fast and accurate data in a true multi-element
analysis could significantly advance further research, for example
in larger studies to assess biological variation or occupational
health.
Evaluation of long-term robustness
To simulate high-throughput analysis of a large number of
samples, a batch of samples containing the 50-fold diluted whole
blood sample solutions previously analyzed were scheduled for
analysis (Figure 3).
After generating calibration standard curves, the batch contained
several blocks of the whole blood samples together with the
required QC checks. The total number of analyses was 287
(including 180 whole blood matrix samples and 28 calibrants and
54 QC checks), requiring a total analysis time of approximately
15 hours.
The regular CCV checks (n=9) indicated excellent recovery
(within 88–124%) for all 43 elements. The iCAP MTX ICP-MS
therefore allows for robust and reliable long-term analysis even
for challenging matrix types like whole blood.
The response of the internal standards is shown in Figure 4. All
internal standards showed reliable and predictable recovery
(within approximately 75% to 112%) over the entire runtime of the
batch, demonstrating robust analytical performance.
Figure 4. Response of the internal standards in a batch covering about ~15 hours of uninterrupted analysis of 287 samples
Figure 3. Schematic overview of the batch analyzed for testing
the long-term performance of the proposed method. Nine blocks,
containing 20 whole blood samples each, were analyzed.
7
Table 5. Quantification results for different whole blood samples. All concentrations are reported as µg·L-1.
MDL Mean horse
(n=9)
RSD
(%)
Mean human
(n=9)
RSD
(%)
Mean pig
n=9)
RSD
(%)
7
Li 0.43 23.2 2.0 0.6 15.5 1.4 7.2
9Be 0.71 <MDL N.D. <MDL N.D. <MDL N.D.
11B 3.41 56.8 8.8 37.7 5.2 47.8 2.5
23Na 31.8 2,433,814 0.7 1,986,726 0.4 3,069,314 0.8
24Mg 2.43 37,045 0.5 31,892 0.7 54,173 0.4
27Al 0.81 5.0 11.3 5.0 4.7 8.4 5.2
28Si 8.65 989.1 1.9 557.1 1.9 487.4 0.7
31P 13.5 248,530 0.5 353,021.9 0.6 550,665 0.8
33S 361.2 1,128,097 0.6 1,436,586 0.9 1,018,857 0.2
39K 5.10 2,157,418 0.9 2,357,705 0.6 2,084,854 0.7
44Ca 79.6 99,752 0.5 62,057 1.0 60,445 0.5
49Ti 0.52 3.5 6.8 1.5 7.6 1.9 5.4
51V 0.04 0.3 7.7 0.1 6.1 0.8 2.2
52Cr 0.66 1.4 9.8 0.8 15.2 1.2 8.3
55Mn 0.20 6.2 0.9 6.2 1.4 13.8 0.7
57Fe 118.0 306,624 0.7 342,230 0.3 360,384 0.5
59Co 0.03 0.6 2.2 0.6 1.2 0.2 5.7
60Ni 0.32 0.9 11.4 2.7 3.6 <MDL N.D.
63Cu 0.47 787.5 0.3 1,127 0.3 1,061 0.4
66Zn 1.76 1,998 0.3 4,476 0.3 3,089 0.3
71Ga 0.24 <MDL N.D. <MDL N.D. <MDL N.D.
75As 0.48 0.6 0.5 5.0 1.0 <MDL N.D.
80Se 0.50 200.2 1.1 113.7 0.9 215.6 0.4
85Rb 0.03 1,933 0.3 1,495 0.1 2,233 0.8
88Sr 0.14 55.1 0.9 15.9 0.9 58.4 0.5
90Zr 0.12 <MDL N.D. <MDL N.D. 25.3 1.3
93Nb 0.02 <MDL N.D. <MDL N.D. <MDL N.D.
95Mo 0.42 3.5 1.9 1.2 5.2 5.9 1.9
107Ag 0.12 0.76 9.8 0.3 11.2 0.6 9.5
111Cd 0.43 <MDL N.D. <MDL N.D. <MDL N.D.
115In 0.05 <MDL N.D. <MDL N.D. <MDL N.D.
118Sn 0.07 <MDL N.D. <MDL N.D. <MDL N.D.
121Sb 0.20 3.4 3.4 <MDL N.D. <MDL N.D.
137Ba 0.40 3.6 12.0 1.4 10.8 7.5 3.3
181Ta 0.01 <MDL N.D. <MDL N.D. <MDL N.D.
182W 0.02 <MDL N.D. <MDL N.D. <MDL N.D.
185Re 0.02 <MDL N.D. <MDL N.D. <MDL N.D.
195Pt 0.12 <MDL N.D. <MDL N.D. <MDL N.D.
202Hg 0.12 0.5 3.8 1.1 2.3 0.5 2.4
205Tl 0.04 <MDL N.D. <MDL N.D. <MDL N.D.
208Pb 0.05 2.9 5.5 5.7 0.9 1.5 3.5
209Bi 0.03 <MDL N.D. <MDL N.D. <MDL N.D.
238U 0.02 <MDL N.D. <MDL N.D. 0.1 7.7
8
General Laboratory Equipment – Not For Diagnostic Procedures. © 2024 Thermo Fisher Scientific Inc.
All rights reserved. All trademarks are the property of Thermo Fisher Scientific and its subsidiaries unless otherwise specified.
Savillex is a trademark of Savillex, LLC. Triton-X and Sigma-Aldrich are trademarks of Merck KGaA. Seronorm is a trademark of
SERO AS. This information is presented as an example of the capabilities of Thermo Fisher Scientific products. It is not intended to
encourage use of these products in any manner that might infringe the intellectual property rights of others. Specifications, terms and
pricing are subject to change. Not all products are available in all countries. Please consult your local sales representative for details.
TN003015-EN 0624S
Learn more at thermofisher.com/icp-ms
• The developed method provides the required detection
limits and a linear response for all analytes, which cover a
concentration range of 8 orders of magnitude (from sub ng·L-1
to 50 mg·L-1).
• The iSC-65 Autosampler equipped with the Step Ahead
feature allowed for a 9% reduction of the total analysis time,
and in combination with intelligent Matrix Handling, the
analysis of whole blood could be carried out with improved
productivity.
• The use of TQ-O₂ mode allowed removal of interferences
of any kind, especially on key analytes such as common
transition metals, and toxic/essential elements such as arsenic
or selenium in the 50-fold diluted whole blood samples. This
is proven by the excellent results obtained for the analysis of
whole blood reference materials.
• The total analysis time was 3 min 14 s per sample (including
uptake and wash time) for 43 elements (covering major,
essential, and trace level analytes). This is specifically for
large cohort studies, for example to screen exposure in a
representative population. The analysis time could be further
shortened with a discrete sampling valve.
• Robust and stable analytical performance was demonstrated
over 15 hours of continuous acquisition of 287 samples by the
iCAP MTX ICP-MS with excellent CCV results.
References
1. Simic, A. et al. Trace elements in whole blood in the general population in Trøndelag
County, Norway: The HUNT3 Survey. Science of the Total Environment, 2022, 806,
150875.
2. Thermo Fisher Scientific. Product Spotlight 44485: Thermo Scientific iCAP Qnova
Series ICP-MS PLUS Torch for Improved ICP-MS Analysis of Challenging Samples.
3. Thermo Fisher Scientific. Technical note 43283: Total elemental analysis in clinical
research using the Thermo Scientific iCAP TQ ICP-MS.
Figure 5 shows an image of the sample and skimmer cone after
aspirating over 1,000 whole blood samples tested in this study.
No deposition of material on the cone surface was observed;
thus, this analysis configuration enables the analysis of whole
blood solutions over longer periods.
Conclusions
The iCAP MTX ICP-MS was employed to analyze 43 elements
in whole blood samples. Among the analytes, several critical
interferences can cause unexpected bias, which were
investigated closely for effective and complete removal by means
of selective collision / reaction cell reactions with oxygen. This
analytical method was rigorously tested, and the results obtained
clearly demonstrated the following analytical advantages:
• The combination of He KED and TQ-O2
mode allows for high
sensitivity analysis required for the accurate determination of
the entire mass range (lithium to uranium), while effectively
suppressing typical interferences.
Figure 5. Sample (A) and skimmer (B) cone condition after running
more than 1,000 whole blood samples
Brought to you by
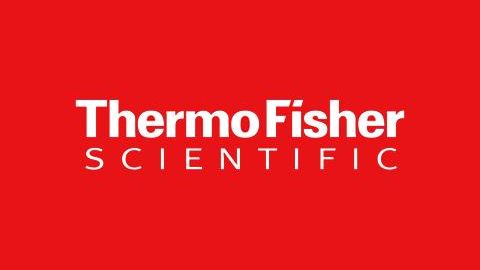
Download the Application Note for FREE Now!
Information you provide will be shared with the sponsors for this content. Technology Networks or its sponsors may contact you to offer you content or products based on your interest in this topic. You may opt-out at any time.
Experiencing issues viewing the form? Click here to access an alternate version