Achieve Streamlined VOC Analysis in Ambient Air
App Note / Case Study
Published: January 27, 2025
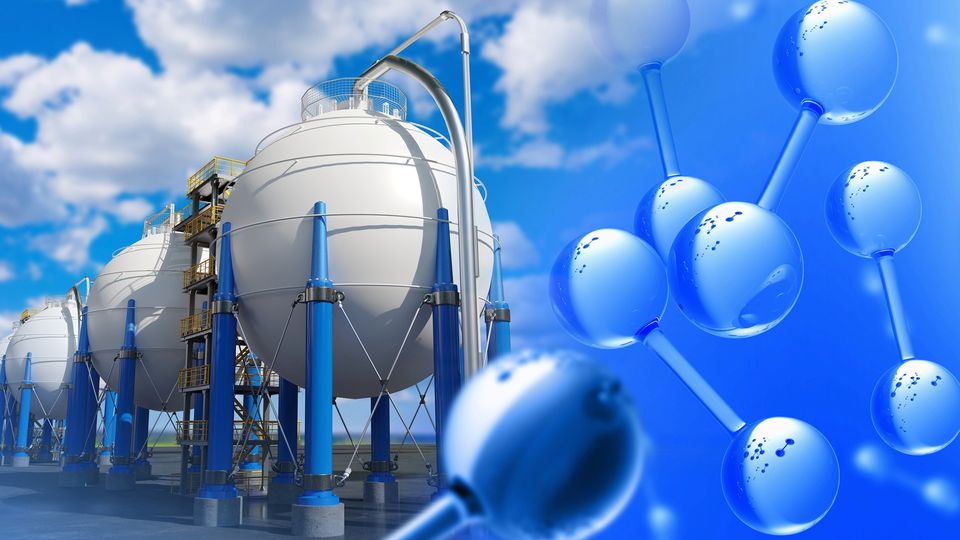
Credit: iStock
Volatile organic compounds (VOCs) play a critical role in environmental monitoring and semiconductor manufacturing.
Proper analysis and control of VOCs are essential for regulatory compliance, maintaining cleanroom standards and ensuring product quality.
This application note outlines a robust methodology for analyzing 65 VOCs in ambient air using canister sampling combined with thermal desorption-gas chromatography/mass spectrometry (TD-GC/MSD).
Download this application note to learn how to:
- Achieve exceptional sensitivity and precision in VOC detection
- Ensure compliance with the latest environmental monitoring and cleanroom standards
- Leverage a reliable and efficient workflow for analyzing complex VOC compositions in ambient air
Application Note
Semiconductor and
Environmental
Authors
Youjuan Zhang and Yu-tian Xu
Agilent Technologies
(Shanghai) Co. Ltd.
Jun Lu
Markes International
Shanghai, China
Abstract
This application note introduces the use of a canister for gas sampling. Samples
were introduced into an Agilent 8890 gas chromatograph (GC) with an Agilent 5977
single quadrupole mass selective detector (MSD) and the Markes Multi-Gas thermal
desorption (TD), to analyze volatile organic compounds (VOCs) in the atmosphere or
in semiconductor cleanrooms. The results of linearity, reproducibility, and detection
limit fully met the requirements of the China method HJ759-2023. This method is
widely used as a reference both in environmental laboratories and semiconductor
cleanrooms. The linear correlation coefficient for all 65 compounds was greater
than 0.991, with 86% of compounds having a value greater than 0.999. The results
of reproducibility in scan mode varied between 0.3 to 6.8%, while results in SIM
mode varied between 1.1 to 6.7%. The sampling volume was 300 mL, which
resulted in detection limits of 0.013 to 0.113 nmol/mol for scan mode and 0.002 to
0.013 nmol/mol for SIM mode.
Analysis of 65 Volatile Organic
Compounds in Ambient Air by
Canister Sampling Using an
Agilent 8890/5977 GC/MSD
A method using both scan and SIM mode that fully
meets the requirements in the HJ 759-2023 standard
2
Introduction
VOCs are a common type of organic pollutant that are
highly irritating and harmful, with a significant impact on the
environment. The composition of VOCs in the atmospheric
environment is complex and they are crucial contributors
to the formation of photochemical smog in the air. The
study of VOCs has become a focus both domestically
and internationally, with many countries issuing a series
of regulations for the monitoring of air quality. In China,
Europe, America, and other places, the detection of VOCs in
the environment is not only a key project for city, regional,
or national environmental monitoring stations, but also for
certain manufacturing enterprises. These manufacturers,
such as wafer factories, require strict control of VOCs
in cleanrooms. Currently, with the rapid development of
semiconductor technology and chip production entering the
nanometer scale, the corresponding requirements for the
production environment are becoming successively greater.
The control of molecular-level air pollutants in cleanroom
environments is becoming increasingly urgent, and VOCs
are one of the molecular-level air pollutants that need to be
strictly controlled. The main sources of VOCs in cleanrooms
include interior decoration materials, equipment materials,
cleaning agents, outdoor air, etc.1
VOC pollutants in the
ambient air can cause contamination on the surface of
semiconductor wafers, corrosion of connecting wires, and
other problems that endanger product quality. Therefore, VOC
pollutants have become a major factor affecting industrial
development of wafers and their yield rate.
In the analysis of VOCs in ambient air, thermal desorption
gas chromatography/mass spectrometry (TD-GC/MSD) is
mostly used for detection both in environmental monitoring
labs and semiconductor industry cleanrooms. There are two
common ways to collect samples. One type of sampling
involves the use of an adsorption tube for sample collection
and preconcentration, followed by analysis through a
TD-GC/MSD system. The HJ 734-20142
method specifies the
use of adsorption tubes and TD-GC/MSD to detect 24 VOCs.
Markes International has published an application note based
on the HJ734 method, which shows excellent performance
results using adsorption tube sampling method.3
An
alternative method employs a pre-evacuated canister for
sample collection, with low-temperature condensation used
for preconcentration. The HJ759-20234 standard provides a
detailed description of a method using a canister for sampling
and determining 65 VOCs in ambient air.
This study adopts the HJ759-2023 method as a reference,
using canister sampling for the qualitative and quantitative
analysis of 65 VOCs. Excellent linearity, high precision, and
excellent reproducibility results were demonstrated, which
shows the inertness of the full flow path, high sensitivity, and
durability of the whole TD-GC/MSD system.
Experimental
This study was performed on an 8890 GC coupled to a
5977 single quadrupole GC/MSD with an electron ionization
(EI) source. A Markes Multi-Gas thermal desorption sample
introduction and preconcentration system was used and
included three modules:
– CIA Advantage-xr (CIA): canister autosampler
– Kori-xr: water removal module
– Unity-xr: thermal desorption instrument
First, three internal standards (ISTDs) were introduced
into the Unity trap by CIA. Then, the sample was passed
through a heated transfer line through the CIA Advantage
into the Kori for water removal. Finally, the sample flowed
into the focusing cold trap of the Unity for preconcentration.
Eventually, the Unity trap was desorbed at high temperature,
and the 65 VOCs and three ISTDs all entered the GC/MSD
for analysis. Figure 1 shows the flow path of the combined
system. Tables 1 and 2 illustrate the instrument conditions
and consumables used in the system.
Figure 1. Schematic illustration of the flow path of a Markes TD and an
Agilent 8890/5977 GC/MSD system.
TD system GC system
Split
tube
Agilent 8890 GC
Agilent DB-624UI,
60 m × 0.25 mm, 1.4 μm
Standard ISTD
gas
Kori trap
Unity trap
Agilent
5977 MSD Stream
selection
3
In this study, gas standards were purchased from Zhongce
Standards Technology Co., Ltd. (Chengdu, China). One
cylinder contained a mixed standard of 65 VOCs, each
with a concentration approximating 1 µmol/mol. The other
cylinder contained four compounds, three of which were
ISTDs (bromochloromethane: ISTD 1; 1,4-difluorobenzene:
ISTD 2; and chlorobenzene-d5
: ISTD 3); the fourth compound
was 4-bromofluorobenzene (BFB). All had concentrations of
approximately 1 µmol/mol. BFB was required for injection
during the tuning evaluation to learn if the entire system,
particularly the mass spectrometer, was operating at optimal
conditions. These two standard gas cylinders both used
nitrogen as the balance gas.
Preparation of 65 VOCs standard gas
Before establishing the calibration curve, the 1 µmol/mol
standard gas had to be diluted to 0.5, 5, and 20 nmol/mol,
respectively, and stored in canisters. According to the
requirements of the HJ 759 method, to simulate real samples
as much as possible, the standard gas also needed to
be humidified. The relative humidity of the standard gas
in canisters after final dilution was 50%. Therefore, after
cleaning canisters, a certain amount of deionized water
was added to the canisters according to the humidification
method in Appendix B of HJ 759. Then, through a static
dilution system using high-purity nitrogen (99.999% purity)
as the dilution gas, the 1 µmol/mol standard gas was
diluted to the target concentration levels. As shown in
Tables 3 and 4, in scan mode, the target concentrations
were 5 and 20 nmol/mol, respectively. In SIM mode, the
target concentrations were 0.5 and 5 nmol/mol, respectively.
Then different volumes were pumped in by the CIA to obtain
calibration levels with different concentrations.
Scan 5 nmol/mol 20 nmol/mol
Sample Volume (mL) 30 60 150 60 150 300
Calibration Levels (nmol/mol) 0.5 1 2.5 4 10 20
Table 3. Preparation of calibration mixture of 65 VOCs for scan mode.
SIM 0.5 nmol/mol 5 nmol/mol
Sample Volume (mL) 60 150 300 60 150 300
Calibration Levels (nmol/mol) 0.1 0.25 0.5 1 2.5 5
Table 4. Preparation of calibration mixture of 65 VOCs for SIM mode.
Table 1. TD parameters.
Parameter Value
Instrument Multi-Gas UNITY-xr, Multi-Gas CIA
Advantage xr, Kori-xr
Cold Trap Markes HJ 759 (p/n U-HJ759-KXR)
General
Flow Path Temperature 120 °C
Sampling Line Temperature 120 °C
Presampling
Sample Purge Time 0.1 min
Sample Purge Flow 50 mL/min
Internal Standard Volume 30 mL
Sampling
Sample Volume 30 to 300 mL
Sampling Flow 20, 50 mL/min
Post Sampling Purge
Post Sampling Purge Time 4 min
Post Sampling Purge Flow 50 mL/min
CIA Post Sampling Purge Flow 50 mL/min
Kori Settings
Kori Trap Low –30 °C
Kori Trap High 300 °C
Trap Settings
Trap Purge Time 1 min
Elevated Trap Purge Temperature 10 °C
Trap Purge Flow 50 mL/min
Trap Low Temperature –25 °C
Trap High Temperature 250 °C
Trap Desorption Time 3 min
Desorb Split Flow 2 mL/min
Table 2. GC conditions.
Parameter Value
Agilent 8890 GC
Column Agilent DB-624 UI, 60 m × 0.25 mm, 1.4 μm
(p/n 122-1364 UI)
Carrier Gas Helium, constant flow, 1 mL/min
Oven Program 35 °C (5 min), 5 °C/min to 150 °C (7 min),
10 °C/min to 200 °C (4 min)
Agilent 5977 MSD
Ion Source Temperature 230 °C
Quad Temperature 150 °C
Tune File Atune.u
Acquisition Type Scan/SIM, m/z scan range 35 to 500
Gain Factor 1
Drawout Plate 6 mm
4
Preparation of ISTD gas
The 1 µmol/mol ISTD was directly diluted to 50 nmol/mol
with high purity nitrogen. The sample volume pumped into
the system was 300 mL, as detailed in the HJ 759 method.
By pumping 30 mL into the system through the CIA, the
corresponding concentration of the internal standards
distributed in a 300 mL sample was 5 nmol/mol.
The HJ 759 method requires the use of canisters for sample
collection. Some of the 65 VOCs have high polarity and were
easily adsorbed; it was therefore necessary to use passivated
canisters. Moreover, the canisters had to rigorously be
cleaned before use to reduce their own adsorption of polar
or high-boiling point compounds. It is suggested to prepare a
canister filled with high purity nitrogen. Before each batch of
samples is run, a blank check of the canister and the system
should be carried out.
Results and discussion
TD parameter optimization
To simulate the humidity of real samples, the standard gas
was also subjected to 50% humidification treatment during
preparation. The UNITY-CIA Advantage-xr Kori-xr instrument
employs Dry Focus3 for effective management of humidity
from gas phase samples, ensuring robust results, extending
the life of the GC column and prolonging source cleaning of
the MSD.
Dry Focus3 is a fully automated three-stage process for
drying, focusing, and injecting humid samples. Figure 2
shows the three-stage process. Step 2 takes advantage of a
temperature-programmed purge of the cold trap to eliminate
residual water retained by the strong sorbents required for
HJ 759. Even small amounts of residual water can affect the
Figure 2. The schematic shows the whole process of Dry Focus3.
Step 1 – Sampling
The sample passes through a drying trap to selectively
remove water before concentration of the analytes on the
focusing trap.
Step 2 – Trap purge
A temperature-programmed purge of the focusing trap
allows elimination of residual water while retaining all
trapped analytes.
Step 3 – Desorption
The focusing trap heats rapidly in a reversed flow of carrier
gas to inject analytes into the GC column. At the same time,
the drying trap is regenerated for the next sample.
Kori-xr trap
Focusing trap
Carrier gas
To vent
To GC
Sample
5
ionization efficiency of the mass spectrometer, impacting
target compounds that coelute with water to the greatest
degree. In this study, analyte trapping at –25 °C ensured
sensitive detection of target compounds while an elevated
temperature purge was optimized to eliminate residual water.
The higher the purge temperature and the longer the time, the
sooner the more volatile targets will begin to breakthrough
to be lost from the Unity trap. If the purge temperature is too
low or the time is too short, effective water removal is not
achieved. Optimized elevated temperature purge conditions
of 10 °C and 50 mL/min purge flow for 1 minute are shown in
Figures 3 and 4; this balances retention of the most volatile
target analytes with efficient water removal.
Figure 3. Comparison of chromatograms in scan mode (scan from m/z 35) with and without "enable elevated trap purge temperature" function. For the water
check, scan from m/z 12.
1,3-Butadiene
Chloroethene
Without elevated trap purge temperature
Elevated trap purge temperature at 10 °C for 1 minute
Acquisition time (min)
5.0 5.5 6.0 6.5 7.0 7.5 8.0 8.5 9.0 9.5 10.0 10.5 11.0 11.5 12.0 12.5
Significant reduction in water reaching the mass spectrometer
with optimized Dry Focus3 conditions.
m/z 18
5.2 5.4 5.6 5.8 6.0 6.2 6.4
Figure 4. Enable the "elevated trap purge temperature" function in TD software.
6
Scan mode results
Scan mode is used for combined qualitative analysis of
unknown compounds along with targeted quantitative
analysis. With the library search function, the mass spectrum
of the unknown peak can be compared with the standard
mass spectrum in the library, providing preliminary qualitative
results. Figure 5 is the total ion chromatogram (TIC) of
2.5 nmol/mol standard gas obtained under the full scan mode
for 65 VOCs. All compound peaks are sharp and symmetrical.
For some polar compounds, such as 1,4-dioxane and
isopropanol, the peak shape remains outstanding even when
the relative humidity of the sample is 50%. Most compounds
have achieved baseline separation, while a small number of
the compounds are coeluted. Because different quantitative
ions can be selected during quantification, it does not affect
accurate qualitative and quantitative analysis. In Figure 5, in
addition to the 65 VOCs and three ISTDs, there is also a BFB
peak. This is because BFB was prepared with three internal
standard substances in a standard gas cylinder, so the peak
of BFB can be seen in scan mode. In contrast, in SIM mode,
only the ions of the target substance and the ISTD substance
were collected, so the BFB peak could not be found in the TIC
in SIM mode (Figure 7).
The calibration curves were developed based on the ISTD
method for six concentration levels from 0.5 to 20 nmol/mol.
The 6 mm drawout plate was used for the best linearity
for all compounds. For each component at every level of
calibration, an average relative response factor (RRF) was
determined with the ISTD method. The RRF for the percent
relative standard deviation (%RSD) was less than 28% for all
substances analyzed. In relation to linear curve fitting, the
correlation coefficient (R2
) was found to be at least 0.994 for
each component, as shown in Table 5.
Repeatability was assessed by calculating the relative
standard deviation (RSD) of the area from eight replicate
runs at 0.5 nmol/mol (low), 2.5 nmol/mol (middle), and
10 nmol/mol (high) concentration levels (Figure 6). Table 5
shows the overall area %RSD was 0.3 to 6.8 %. For middle and
high concentration levels, the area %RSD of most compounds
was less than 3%. For low concentration, due to the smaller
peak area, the RSD would be slightly larger, with the %RSD of
most compounds ranging between 3 to 4%.
In this study, the method detection limit (MDL) was
established by conducting eight replicated tests using a
0.5 nmol/mol standard gas. The concentration of each
compound was calculated using a linear equation, followed
by the calculation of the standard deviation and multiplication
by 3 to determine the MDL. The study showed that the
calculated detection limits for 65 VOCs ranged from 0.013 to
0.113 nmol/mol, as shown in Table 5.
Figure 5. Total ion chromatogram of 65 VOCs at the concentration of 2.5 nmol/mol in scan mode
×105
0
0.1
0.2
0.3
0.4
0.5
0.6
0.7
0.8
0.9
1.0
1.1
1.2
1.3
1.4
1.5
1.6
4 6 8 10 12 14 16 18 20 22 24 26 28 30 32 34 36 38 40 42
1
2
3
4
5
6 7
8
9
10
11,12
13
14,15
16
17,18
19
20
21
22,23
24
25,26
27
28
29
30,31
32
33
34
35
37
36
38
39
40
41
42
43
44
45
46
47
48
49
50,51
52,53
54
55
56
57
58 59 60
61
62
63
64
65
BFB
ISTD3
ISTD2
ISTD1
7
Table 5. Linearity, repeatability, and MDL results for 65 VOCs in scan mode (continued on the next page).
No. Name RT m/z
RRF
%RSD CF R2
Area %RSD MDL
Low Mid High (nmol/mol) ISTD
1 Propene 4.813 41 5.7 0.9984 1.9 1.5 1 0.039 1
2 Dichlorodifluoromethane 4.929 85 3.1 0.9996 2.9 0.9 1 0.032 1
3 1,1,2,2-Tetrafluoro-1,2-dichloroethane 5.36 134.9 3.3 0.9993 2.7 1.2 1 0.041 1
4 Chloromethane 5.515 50 8.1 0.9956 6.3 2.5 1 0.113 1
5 Chloroethene 5.921 62 3.4 0.9996 2.7 0.8 1 0.040 1
6 1,3-Butadiene 6.045 54 4.3 0.9997 5.1 1.9 1 0.072 1
7 Bromomethane 7.00 94 7.2 0.9941 5.1 0.8 1 0.069 1
8 Chloroethane 7.331 64 3.6 0.9997 4.4 1.7 1 0.056 1
9 Trichlorofluoromethane 8.134 101 3.6 0.9995 2.3 0.3 1 0.039 1
10 Acrolein 9.325 56.1 8.6 0.9997 6.6 2 1 0.067 1
11 1,1-Dichloroethylene 9.63 96 5.0 0.9997 3.6 1.4 1 0.050 1
12 1,2,2-Trifluoro-1,1,2-trichloroethane 9.671 150.9 2.8 0.9995 2.9 1.3 1 0.047 1
13 Acetone 9.762 58 2.2 0.9999 4.1 1.5 1 0.064 1
14 Isopropyl alcohol 10.187 45 7.5 0.9988 4.6 1.3 1 0.056 1
15 Carbon disulfide 10.236 76 3.1 0.9997 2 0.8 1 0.023 1
16 Dichloromethane 10.937 84 3.8 0.9996 3.4 1.6 1 0.041 1
17 trans-1,2-Dichloroethylene 11.693 96 4.3 0.9996 5.1 1.6 1 0.072 1
18 Methyl tert-butyl ether 11.73 73 9.0 0.9997 3.1 1.6 1 0.033 1
19 n-Hexane 12.435 57 6.1 0.9997 2.8 1.4 1 0.033 1
20 1,1-Dichloroethane 12.766 63 3.9 0.9995 2 1.4 1 0.039 1
21 Vinyl acetate 12.9 43 13.2 0.9996 4.3 1.3 1 0.044 1
22 cis-1,2-Dichloroethylene 14.246 96 4.1 0.9997 2 1.3 1 0.027 1
23 2-Butanone 14.272 72 8.6 0.9997 6.8 1.3 1 0.068 1
24 Ethyl Acetate 14.433 43 10.1 0.9998 5.3 2 1 0.059 1
25 Trichloromethane 15.02 83 4.5 0.9998 1.7 0.7 2 0.041 2
26 Tetrahydrofuran 15.038 72 12.3 0.9997 5.8 1.3 1 0.058 1
27 1,1,1-Trichloroethane 15.548 97 3.1 0.9998 3.3 0.7 2 0.048 2
28 Cyclohexane 15.721 84 5.9 0.9997 2.8 1.9 2 0.027 2
29 Carbon Tetrachloride 15.983 116.9 3.3 0.9997 3 1.1 2 0.031 2
30 1,2-Dichloroethane 16.471 62 4.4 0.9999 3.1 1.2 2 0.048 2
31 Benzene 16.471 78 5.3 0.9998 1.2 0.5 2 0.031 2
32 Heptane 17.117 43 6.8 0.9998 4.1 1.4 2 0.046 2
33 Trichloroethylene 18.081 130 5.9 0.9999 2.9 1.1 2 0.042 2
34 1,2-Dichloropropane 18.627 63 2.7 0.9999 3.3 1.7 2 0.039 2
35 Methyl methacrylate 18.888 100.1 17.4 0.9991 4.8 1.7 2 0.058 2
36 1,4-Dioxane 18.995 88 11.3 0.9951 3.5 2.3 2 0.058 2
37 Bromodichloromethane 19.296 83 4.0 0.9999 3.1 1.1 2 0.041 2
38 cis-1,3-Dichloropropene 20.459 75 6.7 0.9996 3 0.7 2 0.045 2
39 Dimethyl disulfide 20.709 94 17.2 0.9978 3.8 2.1 2 0.027 2
40 Methyl isobutyl ketone 20.839 43 8.6 0.9991 3.2 1.7 2 0.040 2
41 Toluene 21.376 91 4.4 0.9999 1.4 1.1 2 0.027 2
42 trans-1,3-Dichloropropene 21.882 75 8.5 0.9997 2.9 1.2 2 0.036 2
43 1,1,2-Trichloroethane 22.38 96.9 3.2 0.9997 1.7 1.1 2 0.032 2
44 Tetrachloroethylene 22.863 165.8 5.6 0.9997 1.6 0.8 2 0.046 2
45 2-Hexanone 23.042 43 12.4 0.9989 3.7 1.2 2 0.047 2
8
No. Name RT m/z
RRF
%RSD CF R2
Area %RSD MDL
Low Mid High (nmol/mol) ISTD
46 Dibromochloromethane 23.467 128.8 4.5 0.9998 1.7 1 2 0.029 2
47 1,2-Dibromoethane 23.815 106.9 3.9 0.9999 2.6 1.8 2 0.041 2
48 Chlorobenzene 25.169 112 3.2 0.9999 1.4 1.2 3 0.019 3
49 Ethylbenzene 25.455 91 6.9 0.9998 2.5 0.8 3 0.020 3
50,51 m,p-Xylene 25.778 91 8.9 0.9998 2.4 0.8 3 0.013 3
52 o-Xylene 26.898 91.1 9.3 0.9998 3.1 0.8 3 0.027 3
53 Styrene 26.924 104 15.3 0.9997 3.4 0.4 3 0.027 3
54 Bromoform 27.449 173 6.2 0.9997 3.2 1.3 3 0.036 3
55 1,1,2,2-Tetrachloroethane 28.735 83 6.4 0.9997 3.3 1.1 3 0.034 3
56 p-Ethyltoluene 29.528 105.1 17.6 0.9996 1.8 1.7 3 0.016 3
57 1,3,5-Trimethylbenzene 29.729 105.1 19.6 0.9997 3.1 1.7 3 0.024 3
58 1,2,4-Trimethylbenzene 31.029 105 22.3 0.9996 4.7 1.3 3 0.029 3
59 1,3-Dichlorobenzene 32.115 146 8.4 0.9998 3.4 1 3 0.032 3
60 1,4-Dichlorobenzene 32.46 146 9.3 0.9998 3.8 0.9 3 0.032 3
61 Benzyl chloride 32.984 91 27.8 0.9988 3.2 1.4 3 0.024 3
62 1,2-Dichlorobenzene 34.065 145.9 10.3 0.9997 3.6 0.6 3 0.036 3
63 1,2,4-Trichlorobenzene 40.312 179.9 11.4 0.9992 4.7 2.6 3 0.054 3
64 Hexachlorobutadiene 40.908 224.8 11.3 0.9996 4.6 1.4 3 0.046 3
65 Naphthalene 41.101 128 22.4 0.9986 4.9 2.4 3 0.042 3
Area %RSD
0
2
4
6
8
10
12
14
Propene
Dichlorodifluoromethane
1,1,2,2-Tetrafluoro-1,2-dichloroethane
Chloromethane
Chloroethene
1,3-Butadiene
Bromomethane
Chloroethane
Trichlorofluoromethane
Acrolein
1,1-Dichloroethylene
1,2,2-Trifluoro-1,1,2-trichloroethane
Acetone
Isopropyl alcohol
Carbon disulfide
Dichloromethane
trans-1,2-Dichloroethylene
Methyl tert-butyl ether
n-Hexane
1,1-Dichloroethane
Vinyl acetate
cis-1,2-Dichloroethylene
2-Butanone
Ethyl Acetate
Trichloromethane
Tetrahydrofuran
1,1,1-Trichloroethane
Cyclohexane
Carbon Tetrachloride
1,2-Dichloroethane
Benzene
Heptane
Trichloroethylene
1,2-Dichloropropane
Methyl methacrylate
1,4-Dioxane
Bromodichloromethane
cis-1,3-Dichloropropene
Dimethyl disulfide
Methyl isobutyl ketone
Toluene
trans-1,3-Dichloropropene
1,1,2-Trichloroethane
Tetrachloroethylene
2-Hexanone
Dibromochloromethane
1,2-Dibromoethane
Chlorobenzene
Ethylbenzene
m,p-Xylene
o-Xylene
Styrene
Bromofor
m
1,1,2,2-Tetrachloroethane
p-Ethyltoluene
1,3,5-Trimethylbenzene
1,2,4-Trimethylbenzene
1,3-Dichlorobenzene
1,4-Dichlorobenzene
Benzyl chloride
1,2-Dichlorobenzene
1,2,4-Trichlorobenzene
Hexachlorobutadiene
Naphthalene
0.5 nmol/mol 2.5 nmol/mol 10 nmol/mol
Figure 6. Repeatability results for 65 VOCs at low, middle, and high calibration levels in scan mode.
9
SIM mode results
SIM mode uses a defined compound list to perform sensitive,
targeted analysis. In SIM mode, it is necessary to group the
target substances in advance and input the characteristic
ions of each target substance to establish an acquisition
method. Figure 7 is the TIC of the 1 nmol/mol standard
gas collected under SIM mode. Because only the ions of
interest are collected, only the chromatographic peaks of the
target substances appear on the chromatogram. Therefore,
compared to scan mode, the data collected in SIM mode have
fewer interference peaks, and the BFB peak cannot be seen.
Similar to scan mode data, under SIM mode, all compound
peaks are also sharp and symmetrical, demonstrating the
excellent inertness of the entire TD/GC/MSD system and fast
injection from TD to GC.
In SIM mode, a comprehensive method evaluation was
also performed. The linear range of SIM mode is from 0.1
to 5 nmol/mol, which is approximately 5x lower than the
concentrations in scan mode. Within this linear range, the
RRF %RSD was less than 22% for all substances analyzed.
The correlation coefficient values of 84% of the compounds
were greater than 0.999, while the remaining 16% of the
compounds were all greater than 0.99, which fully meets the
requirements detailed in method HJ759-2023. To verify the
precision of the method and the stability of the instrument,
the concentrations of 0.1 nmol/mol (low), 0.5 nmol/mol
(middle), and 2.5 nmol/mol (high) standard gases were
measured eight times. The peak area %RSD of all compounds
was less than 7%, and the average %RSD value was 2.3%, as
shown in Figure 8. The MDL was determined through eight
replicate runs of a 0.1 nmol/mol standard gas. Under SIM
mode, the MDL values of all compounds ranged from 0.002
to 0.013 nmol/mol, showing the ultrahigh sensitivity of this
system. All the performance results are shown in Table 6.
Figure 7. Total ion chromatogram of 65 VOCs at the concentration of 1 nmol/mol in SIM mode.
×104
4 6 8 10 12 14 16 18 20 22 24 26 28 30 32 34 36 38 40 42
1
2 3
4
5
6 7
8
9
10
11,12
13
14,15
16
17,18
19
20
21
22,23
24
25,26
27
28
29
30,31
32
33
34
35
37
36
38
39
40
41
4243
44
45
4647
48
49
50,51
52,53
54 55
56
57 58 59 60
61
62 63 64
65
ISTD3
ISTD2
ISTD1
0
1
2
3
4
5
6
7
8
9
10
Figure 8. Repeatability results for 65 VOCs at low, middle, and high calibration levels in SIM mode. Area %RSD
0
2
4
6
8
10
12
14
Propene
Dichlorodifluoromethane
1,1,2,2-Tetrafluoro-1,2-dichloroethane
Chloromethane
Chloroethene
1,3-Butadiene
Bromomethane
Chloroethane
Trichlorofluoromethane
Acrolein
1,1-Dichloroethylene
1,2,2-Trifluoro-1,1,2-trichloroethane
Acetone
Isopropyl alcohol
Carbon disulfide
Dichloromethane
trans-1,2-Dichloroethylene
Methyl tert-butyl ether
n-Hexane
1,1-Dichloroethane
Vinyl acetate
cis-1,2-Dichloroethylene
2-Butanone
Ethyl Acetate
Trichloromethane
Tetrahydrofuran
1,1,1-Trichloroethane
Cyclohexane
Carbon Tetrachloride
1,2-Dichloroethane
Benzene
Heptane
Trichloroethylene
1,2-Dichloropropane
Methyl methacrylate
1,4-Dioxane
Bromodichloromethane
cis-1,3-Dichloropropene
Dimethyl disulfide
Methyl isobutyl ketone
Toluene
trans-1,3-Dichloropropene
1,1,2-Trichloroethane
Tetrachloroethylene
2-Hexanone
Dibromochloromethane
1,2-Dibromoethane
Chlorobenzene
Ethylbenzene
m,p-Xylene
o-Xylene
Styrene
Bromofor
m
1,1,2,2-Tetrachloroethane
p-Ethyltoluene
1,3,5-Trimethylbenzene
1,2,4-Trimethylbenzene
1,3-Dichlorobenzene
1,4-Dichlorobenzene
Benzyl chloride
1,2-Dichlorobenzene
1,2,4-Trichlorobenzene
Hexachlorobutadiene
Naphthalene
0.1 nmol/mol 0.5 nmol/mol 2.5 nmol/mol
Table 6. Linearity, repeatability, and MDL results for 65 VOCs in SIM mode (continued on the next page).
No. Name RT
RRF
%RSD CF2
Area %RSD MDL
Low Mid High (nmol/mol) ISTD
1 Propene 4.618 5.0 0.9999 1.9 2.4 2.4 0.004 1
2 Dichlorodifluoromethane 4.729 3.1 0.9999 1.6 1.8 1.9 0.002 1
3 1,1,2,2-Tetrafluoro-1,2-dichloroethane 5.171 2.5 0.9999 1.7 1.9 2.2 0.002 1
4 Chloromethane 5.46 16.0 0.9989 4.1 4.5 3.2 0.013 1
5 Chloroethene 5.725 2.8 0.9999 1.1 2.1 1.9 0.003 1
6 1,3-Butadiene 5.865 2.9 0.9998 2.1 2.1 2.6 0.004 1
7 Bromomethane 6.824 7.0 0.9998 1.9 6.7 1.6 0.004 1
8 Chloroethane 7.149 3.0 0.9998 3.4 2 2.3 0.007 1
9 Trichlorofluoromethane 7.947 3.0 0.9998 1.8 1.9 1.9 0.003 1
10 Acrolein 9.202 10.1 0.9998 3.1 1.7 3.1 0.012 1
11 1,1-Dichloroethylene 9.5 3.4 0.9999 2 2.2 2.7 0.003 1
12 1,2,2-Trifluoro-1,1,2-trichloroethane 9.532 2.9 0.9997 1.7 1.8 1.8 0.004 1
13 Acetone 9.654 21.6 0.9999 2.6 2.9 2.5 0.009 1
14 Isopropyl alcohol 10.081 9.3 0.9981 1.9 2.6 2.4 0.006 1
15 Carbon disulfide 10.105 5.8 0.9999 2 1.6 1.9 0.006 1
16 Dichloromethane 10.824 7.6 0.9998 1.1 1.5 1.9 0.004 1
17 trans-1,2-Dichloroethylene 11.597 3.5 0.9999 1.6 2 2.3 0.003 1
18 Methyl tert-butyl ether 11.648 5.5 0.9996 1.4 2 3.2 0.003 1
19 n-Hexane 12.35 18.5 0.9999 1.7 2.5 3.3 0.007 1
20 1,1-Dichloroethane 12.689 4.0 0.9996 1.5 1.9 2.1 0.002 1
21 Vinyl acetate 12.827 7.9 0.9996 3.4 2.5 3.6 0.008 1
22 cis-1,2-Dichloroethylene 14.177 4.0 0.9998 1.2 2.1 2.4 0.003 1
23 2-Butanone 14.221 5.1 0.9996 3.5 2.1 2.7 0.011 1
24 Ethyl Acetate 14.384 5.7 0.9997 1.6 1.9 2.5 0.005 1
25 Trichloromethane 14.966 3.4 0.9997 1.3 1.9 1.9 0.003 2
11
No. Name RT
RRF
%RSD CF2
Area %RSD MDL
Low Mid High (nmol/mol) ISTD
26 Tetrahydrofuran 15.005 5.7 0.9996 2.2 2.7 2.7 0.007 1
27 1,1,1-Trichloroethane 15.495 2.5 0.9999 1.8 2.2 1.9 0.005 2
28 Cyclohexane 15.665 3.5 0.9999 2.1 2.6 3.1 0.004 2
29 Carbon Tetrachloride 15.932 2.7 0.9998 2 2.5 1.6 0.005 2
30 1,2-Dichloroethane 16.428 8.1 0.9998 2.5 1.9 2 0.007 2
31 Benzene 16.428 6.0 0.9996 2.2 2.4 2.2 0.005 2
32 Heptane 17.079 3.0 0.9997 2.1 2.6 3.3 0.003 2
33 Trichloroethylene 18.041 3.4 0.9998 1.4 2.2 2 0.003 2
34 1,2-Dichloropropane 18.594 3.9 0.9998 1.7 2.2 2.1 0.004 2
35 Methyl methacrylate 18.867 6.7 0.9986 2 2.5 2.9 0.003 2
36 1,4-Dioxane 18.981 15.3 0.9913 2.7 2.4 1.7 0.008 2
37 Bromodichloromethane 19.265 3.4 0.9999 1.7 2.2 1.6 0.004 2
38 cis-1,3-Dichloropropene 20.437 4.8 0.9998 1.9 2.4 2.6 0.002 2
39 Dimethyl disulfide 20.684 10.0 0.9980 2.3 2.7 2.9 0.003 2
40 Methyl isobutyl ketone 20.83 9.1 0.9992 2.4 3.1 2.1 0.004 2
41 Toluene 21.36 5.5 0.9999 2 2.2 2.5 0.004 2
42 trans-1,3-Dichloropropene 21.87 6.2 0.9998 2.3 2.5 2.5 0.005 2
43 1,1,2-Trichloroethane 22.362 4.5 0.9997 1.8 2.2 1.7 0.005 2
44 Tetrachloroethylene 22.851 4.6 0.9997 1.3 2 1.7 0.003 2
45 2-Hexanone 23.04 9.7 0.9993 3.1 3.1 2.1 0.007 2
46 Dibromochloromethane 23.45 4.1 0.9999 1.3 2 1.5 0.003 2
47 1,2-Dibromoethane 23.801 4.1 0.9999 1.4 2.3 1.8 0.003 2
48 Chlorobenzene 25.161 4.4 0.9998 1.2 2.5 2.1 0.003 3
49 Ethylbenzene 25.447 5.4 0.9998 2.5 2.7 2.6 0.005 3
50,51 m,p-Xylene 25.767 9.2 0.9997 2.8 2.7 2.3 0.004 3
52 o-Xylene 26.897 8.9 0.9996 2.8 2.8 2.6 0.005 3
53 Styrene 26.921 13.1 0.9992 2.9 2.7 2.3 0.004 3
54 Bromoform 27.445 5.2 0.9999 2 2.2 1.6 0.004 3
55 1,1,2,2-Tetrachloroethane 28.731 3.3 0.9999 1.6 2.2 1.7 0.003 3
56 p-Ethyltoluene 29.528 11.7 0.9987 2.9 2.5 2.7 0.004 3
57 1,3,5-Trimethylbenzene 29.73 14.4 0.9988 3 2.5 2.5 0.005 3
58 1,2,4-Trimethylbenzene 31.03 13.4 0.9978 2.5 2.6 2.8 0.004 3
59 1,3-Dichlorobenzene 32.113 6.6 0.9999 2.3 2.2 2.2 0.003 3
60 1,4-Dichlorobenzene 32.459 7.6 0.9999 3 2.1 2.4 0.005 3
61 Benzyl chloride 32.993 13.4 0.9978 2.7 2.8 2.6 0.004 3
62 1,2-Dichlorobenzene 34.07 5.9 0.9999 3.1 2.7 2.5 0.006 3
63 1,2,4-Trichlorobenzene 40.318 12.0 0.9992 5.1 3 3.1 0.013 3
64 Hexachlorobutadiene 40.912 7.4 0.9997 2.5 2.8 2.3 0.006 3
65 Naphthalene 41.107 16.5 0.9978 5.8 2.6 4 0.012 3
12
Figure 9. MDL results for 65 VOCs both in scan and SIM mode.
0
0.02
0.04
0.06
0.08
0.10
0.12
Propene
Dichlorodifluoromethane
1,1,2,2-Tetrafluoro-1,2-dichloroethane
Chloromethane
Chloroethene
1,3-Butadiene
Bromomethane
Chloroethane
Trichlorofluoromethane
Acrolein
1,1-Dichloroethylene
1,2,2-Trifluoro-1,1,2-trichloroethane
Acetone
Isopropyl alcohol
Carbon disulfide
Dichloromethane
trans-1,2-Dichloroethylene
Methyl tert-butyl ether
n-Hexane
1,1-Dichloroethane
Vinyl acetate
cis-1,2-Dichloroethylene
2-Butanone
Ethyl Acetate
Trichloromethane
Tetrahydrofuran
1,1,1-Trichloroethane
Cyclohexane
Carbon Tetrachloride
1,2-Dichloroethane
Benzene
Heptane
Trichloroethylene
1,2-Dichloropropane
Methyl methacrylate
1,4-Dioxane
Bromodichloromethane
cis-1,3-Dichloropropene
Dimethyl disulfide
Methyl isobutyl ketone
Toluene
trans-1,3-Dichloropropene
1,1,2-Trichloroethane
Tetrachloroethylene
2-Hexanone
Dibromochloromethane
1,2-Dibromoethane
Chlorobenzene
Ethylbenzene
m,p-Xylene
o-Xylene
Styrene
Bromofor
m
1,1,2,2-Tetrachloroethane
p-Ethyltoluene
1,3,5-Trimethylbenzene
1,2,4-Trimethylbenzene
1,3-Dichlorobenzene
1,4-Dichlorobenzene
Benzyl chloride
1,2-Dichlorobenzene
1,2,4-Trichlorobenzene
Hexachlorobutadiene
Naphthalene
Scan SIM
Figure 9 shows the MDL results calculated under both scan
and SIM modes. Compared to scan mode, the sensitivity
results of SIM mode have improved by approximately 10x,
which is consistent with the expectation. Therefore, if an
analysis involves quantifying known trace components, SIM is
a good choice to enhance sensitivity.
www.agilent.com
DE-000635
This information is subject to change without notice.
© Agilent Technologies, Inc. 2024
Printed in the USA, November 25, 2024
5994-7723EN
Conclusion
An Agilent 8890/5977 GC/MSD system, equipped with a
Multi-Gas CIA Advantage-xr TD provides a robust analytical
procedure for the analysis of 65 VOCs in ambient air.
This system offers a highly efficient and highly sensitive
solution for the simultaneous analysis of multiple VOCs
in both atmospheric quality monitoring laboratories and
semiconductor cleanrooms. Based on different application
requirements, users can choose to collect data in scan or SIM
mode, demonstrating the flexibility of Agilent instruments.
Excellent analytical performance has been achieved based on
this system both in scan and SIM mode, which fully meets the
requirements detailed in method HJ 759-2023.
References
1. GB/T25915.8-2021 Cleanrooms and Associated
Controlled Environments Part 8: Classification of Air
Cleanliness by Chemical Concentration (ACC).
2. HJ 734-2014 Stationary Source Emission – Determination
of Volatile Organic Compounds–Sorbent Adsorption
and Thermal Desorption Gas Chromatography Mass
Spectrometry Method.
3. The Monitoring of Organic Waste Gas VOCs Emitted
by Fixed Pollution Sources is Sampled With Adsorption
Tubes and Analyzed by Thermal Desorption/Gas
Chromatography-Mass Spectrometry Method, Which
Complies with the Chinese Environmental Protection
Standard HJ 734-2014. Agilent Technologies application
note, publication number, 2021.
4. HJ 759-2023 Determination of 65 Volatile Organic
Compounds–Collected in Canisters and Analyzed By Gas
Chromatography/Mass Spectrometry.
Brought to you by
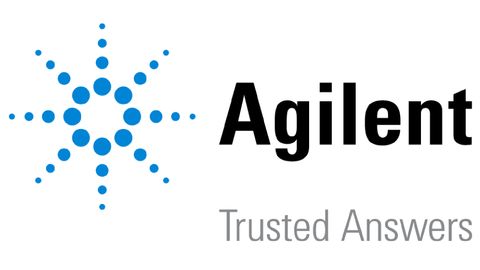
Download the Application Note for FREE Now!
Information you provide will be shared with the sponsors for this content. Technology Networks or its sponsors may contact you to offer you content or products based on your interest in this topic. You may opt-out at any time.
Experiencing issues viewing the form? Click here to access an alternate version