Bacterial Conjugation – Conjugation Biology Explained
Discover the mechanism of conjugation and how scientists use it to manipulate bacterial genomes.
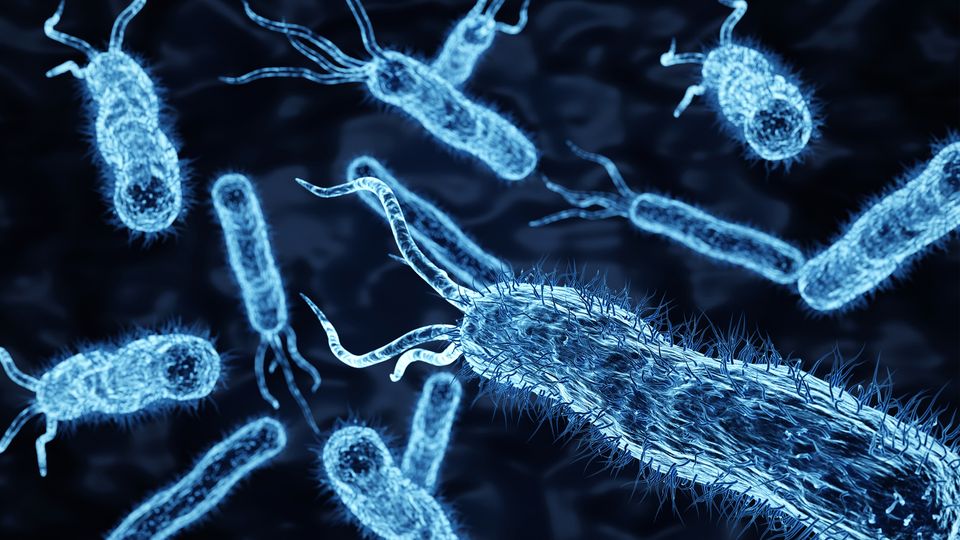
Complete the form below to unlock access to ALL audio articles.
To adapt to changing environmental pressures, bacteria have developed various methods that allow them to uptake exogenous DNA and incorporate it into their own genomes. One of these processes is conjugation, which is often referred to as a type of bacterial parasexual reproduction.
In this article, we will discuss the mechanism of conjugation and how scientists use it to manipulate bacterial genomes.
What is conjugation in biology?
What is conjugation in bacteria?
How does bacterial conjugation work and what's the role of the pilus?
- The mechanism
- F+ conjugation
- Hfr conjugation
- F' conjugation
- Plasmid mobilization
- integrative conjugative elements (ICE)
What is conjugation in biology?
In biology, conjugation is a form of horizontal gene transfer (HGT), along with transformation and transduction, which allows the exchange of genetic material between two organisms or cells. It is observed as a sexual process in eukaryotes, for example, in certain protozoal ciliates.1 However, the process is far more common and has been most extensively studied in bacteria. Conjugation was discovered in the Gram negative bacterium Escherichia coli in 1946 by Joshua Lederberg and Edward Tatum,2 for which Lederberg was awarded a Nobel Prize in physiology and medicine. It is one of the critical mechanisms in the evolution of bacteria and facilitates the spread of traits such as antibiotic resistance, virulence factors and metabolite utilization pathways across populations.
What is conjugation in bacteria?
In classical bacterial conjugation, one bacterium transfers genetic material to another through direct cell-to-cell contact.3 This can either be through a natural process or via artificial means. Natural conjugation is mediated through several methods, for example, conjugative plasmids like the F plasmid, which involves the formation of a sex pilus that allows the transfer of DNA from a donor strain (F+) to a recipient strain (F-). Additionally, integrative and conjugative elements (ICEs) can also initiate conjugation.4 Artificial conjugation is a laboratory process by which scientists enable DNA transfer, for example, using helper plasmids like pRK2013 to mobilize other types of plasmids.5
How does bacterial conjugation work and what’s the role of the pilus?
In general, conjugation involves the transfer of single-stranded DNA (ssDNA), although systems that transfer double-stranded DNA (dsDNA) are known. ssDNA conjugative plasmids tend to be large (> 30kb) and have two sets of components. The first set encodes proteins for mobility (MOB) that allow DNA to be processed and couples this processing with DNA transport. The second set encodes proteins for mating-pair formation (MPF), a type IV secretion system (T4SS) that delivers DNA from the donor to the recipient, of which there are four different types in proteobacteria. There are variations within the systems between bacterial species, but the eventual outcome is the same. Let’s consider the general process in Gram negative bacteria.
The mechanism6,7
1. Conjugation initially requires the expression of the MPF genes in the donor cell. Conjugative T4SS are multi-component protein complexes consisting of four parts: the pilus, the core channel, the inner membrane platform and ATPases that provide the energy for pilus synthesis and DNA transport through the complex. The pilus is the structure that projects from the donor cell into the extracellular environment, contacts recipient cells and then retracts to allow cell-to-cell contact.
2. Once contact between the donor and recipient has occurred, the second step in conjugation is DNA processing. This part of the process is driven by the MOB proteins, of which there are six known families. A relaxome, consisting of a relaxase and other auxiliary proteins, is assembled on the oriT of the plasmid where the relaxase is directed to the nic site (Figure 1A). The relaxase then cleaves the nic site, becomes covalently attached to the DNA to be transferred (T-strand), initiates replication and displaces the T-strand by unwinding it.
3. A T4 coupling protein (T4CP) binds to the relaxome, which is subsequently delivered to the T4SS (Figure 1B) during the process of DNA replication by the relaxase.
4. The relaxase then releases the T-strand by cleaving for a second time at the nic site (Figure 1C). The relaxase is then “shot” through the channel with the T-strand attached, which is then “pumped” through the channel by the T4CP in a process known as “shoot and pump”.
5. Once the T-strand is entirely within the recipient, the relaxase reverses the nicking reaction and generates a complete ssDNA copy of the plasmid (Figure 1D).
6. The final step in the process is to generate a double-stranded version of the plasmid using rolling circle replication (Figure 1E). The recipient cell has now been turned into a new donor.
Conjugation biology diagram
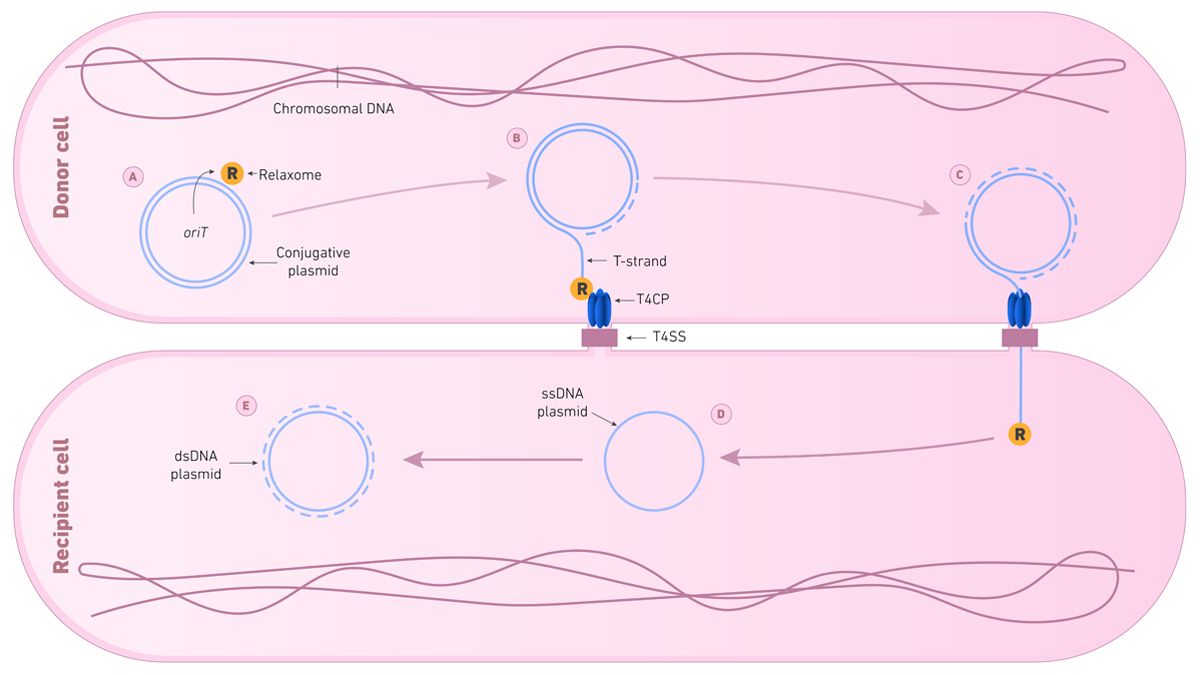
Figure 1: Diagram showing the stages of bacterial conjugation. A) Relaxase in the relaxome complex cleaves the plasmid DNA, generating a T-strand. B) T-strand and relaxome bind TC4P, which is then recruited to the T4SS while DNA replication occurs. C) Relaxome and T-strand are shot and pumped into the recipient cell. D) Plasmid ssDNA is released. E) Plasmid dsDNA is replicated. Credit: Technology Networks.
Types of conjugation
- F+ conjugation
The donor cell is F+ (which contains the F plasmid, which is also known as the fertility factor), and the recipient is F- (which lacks the plasmid). After conjugation, the recipient becomes F+, acquiring the ability to act as a donor in future conjugation events. F+ conjugation is the process described in detail in Figure 1 and was the first system to be described by Lederberg and Tatum.2,8
- Hfr conjugation
Sometimes, the F plasmid can integrate into the donor's chromosome, and the cell becomes a high frequency of recombination (Hfr) cell. During conjugation, portions of the donor's chromosomal DNA adjacent to the integrated plasmid are transferred to the recipient. The transfer is often incomplete, so the recipient remains F- but acquires new chromosomal genes that can be incorporated into its genome.8
- F' conjugation
The F plasmid can excise incorrectly from the donor's chromosome once integrated, and it may carry some chromosomal genes with it, forming an F' plasmid. The recipient gains both the plasmid and the additional chromosomal genes.8
- Plasmid mobilization
Many plasmids are incapable of conjugating on their own (non-conjugative plasmids) as they lack the machinery for conjugation; sometimes, these can be mobilized for transfer by the presence of a conjugative plasmid.9
- Integrative conjugative elements (ICEs)
In addition to plasmids, many bacteria contain ICEs. These are modular mobile genetic elements that combine features of both integrative elements and conjugative plasmids. They can play an important role in HGT by facilitating the exchange of traits such as antibiotic resistance, virulence factors and metabolic capabilities.10
Applications of artificial conjugation in microbiology
The applications of artificial conjugation in microbiology are enormous and range from basic research to highly applied systems designed for specific purposes. One example is the development of systems for manipulating Deinococcus radiodurans, which, due to its innate resistance to various stress-inducing processes, is an attractive option for biotechnological applications like bioremediation.11 Another example is the development of systems to transfer DNA from bacteria to other species like yeast, mammalian cells and plant cells.12,13,14 One final example is the development of synthetic bacteria that use conjugation to kill selected pathogens by transferring toxic genes into them.15
In summary, conjugation is a method of HGT that is critically important to the spread of genetic traits, e.g., antibiotic resistance and virulence factors, within bacterial populations. Additionally, scientists are using artificial conjugation to study gene transfer and manipulate bacterial genomes for a plethora of biotechnological applications.