Protein Lassos Itself, Explaining Its Unusual Behavior
A new mechanism could help explain why some proteins refold in a different pattern than expected.
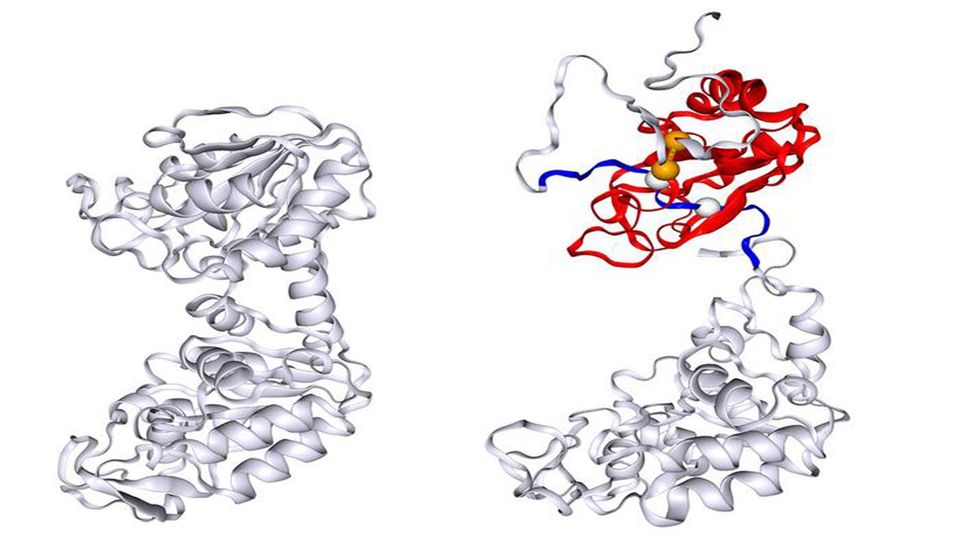
Complete the form below to unlock access to ALL audio articles.
Proteins are long molecules that must fold into complex three-dimensional structures to perform their cellular functions. This folding process occasionally goes awry, resulting in misfolded proteins that, if not corrected, can potentially lead to disease.
Now, a new study has described a potential mechanism that could help explain why some proteins refold in a different pattern than expected. The researchers, led by chemists at Penn State, found that a type of misfolding, in which the proteins incorrectly intertwine their segments, can occur and create a barrier to the normal folding process. Correcting this misfold requires high-energy or extensive unfolding, which slows the folding process leading to the unexpected pattern first observed in the 1990s.
“Misfolded proteins can malfunction and lead to disease,” said Ed O’Brien, professor of chemistry in the Eberly College of Science, a co-hire of the Institute for Computational and Data Sciences at Penn State, and leader of the research team. “So, understanding the mechanisms involved in the folding process can potentially help researchers prevent or develop treatments for diseases caused by misfolding.”
A paper describing the research, which used a combination of computer simulations and refolding experiments to describe the folding kinetics of a protein called phosphoglycerate kinase (PGK), appeared in the journal Science Advances.
Want more breaking news?
Subscribe to Technology Networks’ daily newsletter, delivering breaking science news straight to your inbox every day.
Subscribe for FREE“For most proteins, we model the folding process as if there are two states, folded or unfolded,” said Yang Jiang, assistant research professor of chemistry in the Eberly College of Science at Penn State and the first author of the paper. “When we track the progression of a protein from unfolded to folded, we see a characteristic time-dependent pattern that we call the folding kinetics of the protein. Usually, the proportion of unfolded proteins goes down exponentially until essentially all of the proteins are folded, but some proteins don’t fit this pattern, and we were interested in the mechanisms that might explain this.”
The unusual folding pattern of PGK was first observed experimentally over 25 years ago. Whereas most proteins fit the “two-state” model of exponential folding kinetics, the molecules of PGK followed a different pattern to reach a fully folded state. This new pattern was described as “stretched-exponential refolding kinetics,” but the structural mechanism that explained this difference remained a mystery — until now. The research team hypothesized that a recently described class of misfolding may be responsible for PGK’s deviation from the traditional two-state model of folding.
“Non-covalent lasso entanglement is a class of misfolding we recently identified where a loop of the protein traps another segment of the protein, essentially intertwining itself incorrectly,” O’Brien said. “If a protein like PGK is more prone to this type of misfolding, it could help explain why we see the stretched-exponential refolding kinetics.”
To test this hypothesis, the research team first built a computer model to simulate the folding process of PGK. Their simulations recapitulated the stretched-exponential kinetics seen in the earlier experiments. They then explored the intermediate stages of the folding process in their simulations to see if there were structural changes that could explain the stretched refolding.
“We found several examples of misfolding involving entanglements,” Jiang said. “Sometimes a new entanglement formed and sometimes an entanglement that was part of the protein’s native structure failed to form. In our simulations, we could then remove these misfolding events and saw that the protein folded with the typical two-state exponential pattern.”
To confirm the results of their simulation, the research team, which included experimentalist Stephen Fried and lab members at Johns Hopkins University, examined the structural variation of PGK upon refolding in experiments. They found that the misfolded states predicted in the simulations were consistent with the structural signals experimentally observed in the refolded protein. They also found that these misfolded states were long-lived, suggesting that they are a crucial component of the observed stretched-exponential folding kinetics.
“Because of the nature of this type of misfolding, the protein gets stuck,” Jiang said. “The protein must backtrack in the folding process to correct the mistake, which takes time and is energetically expensive. The demonstration of this mechanism helps expand our understanding of how proteins are folded and gives an example of how it can go wrong. This is basic research, but it could eventually inform how we develop therapeutics for diseases linked to protein misfolding.”
Reference: Jiang Y, Xia Y, Sitarik I, et al. Protein misfolding involving entanglements provides a structural explanation for the origin of stretched-exponential refolding kinetics. Sci Adv. 11(11):eads7379. doi: 10.1126/sciadv.ads7379