Reliable Water Analysis With ICP-MS
App Note / Case Study
Published: March 19, 2025
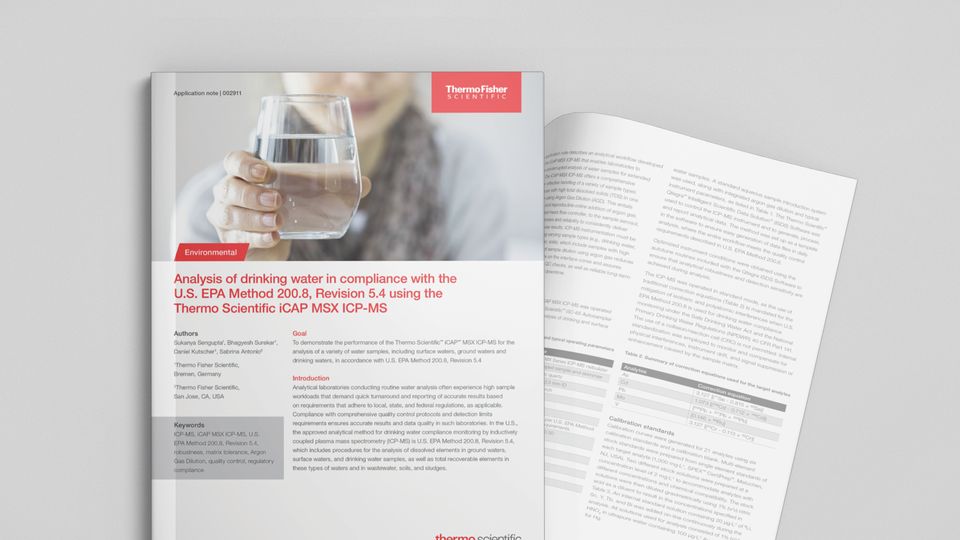
Credit: Thermo Fisher Scientific
Regulatory compliance in water testing requires accurate detection of trace elements across diverse sample types. Yet, laboratories face challenges in ensuring precision and efficiency while adhering to strict quality control standards.
To maintain compliance with the Safe Drinking Water Act, methods must meet stringent detection limits and deliver reproducible results.
This application note highlights an advanced analytical workflow using inductively coupled plasma mass spectrometry (ICP-MS) to streamline drinking water analysis following the US Environmental Protection Agency (EPA) guidelines.
Download this application note to explore:
- A robust ICP-MS workflow that meets the quality control requirements described in EPA Method 200.8, Revision 5.4
- How optimized sample dilution ensures precise detection of trace elements
- Quality control protocols that enhance data reliability and regulatory compliance
Analysis of drinking water in compliance with the
U.S. EPA Method 200.8, Revision 5.4 using the
Thermo Scientific iCAP MSX ICP-MS
Environmental
Application note | 002911
Authors
Sukanya Sengupta1
, Bhagyesh Surekar1
,
Daniel Kutscher1
, Sabrina Antonio2
1
Thermo Fisher Scientific,
Bremen, Germany
2Thermo Fisher Scientific,
San Jose, CA, USA
Goal
To demonstrate the performance of the Thermo Scientific™ iCAP™ MSX ICP-MS for the
analysis of a variety of water samples, including surface waters, ground waters and
drinking waters, in accordance with U.S. EPA Method 200.8, Revision 5.4
Introduction
Analytical laboratories conducting routine water analysis often experience high sample
workloads that demand quick turnaround and reporting of accurate results based
on requirements that adhere to local, state, and federal regulations, as applicable.
Compliance with comprehensive quality control protocols and detection limits
requirements ensures accurate results and data quality in such laboratories. In the U.S.,
the approved analytical method for drinking water compliance monitoring by inductively
coupled plasma mass spectrometry (ICP-MS) is U.S. EPA Method 200.8, Revision 5.4,
which includes procedures for the analysis of dissolved elements in ground waters,
surface waters, and drinking water samples, as well as total recoverable elements in
these types of waters and in wastewater, soils, and sludges.
Keywords
ICP-MS, iCAP MSX ICP-MS, U.S.
EPA Method 200.8, Revision 5.4,
robustness, matrix tolerance, Argon
Gas Dilution, quality control, regulatory
compliance
This application note describes an analytical workflow developed
using the iCAP MSX ICP-MS that enables laboratories to
perform uninterrupted analysis of water samples for extended
periods. The iCAP MSX ICP-MS offers a comprehensive
solution for effective handling of a variety of sample types
including those with high total dissolved solids (TDS) in one
analytical run using Argon Gas Dilution (AGD). This entails
the automatic and reproducible online addition of argon gas,
using a dedicated mass flow controller, to the sample aerosol,
enhancing robustness and reliability to consistently deliver
accurate and precise results. ICP-MS instrumentation must be
capable of analyzing varying sample types (e.g., drinking water,
seawater, wastewater, soils), which include samples with high
TDS. This approach of sample dilution using argon gas reduces
the deposition of matrix on the interface cones and assures
consistent readouts for QC checks, as well as reliable long-term
robustness with minimal downtime.
Experimental
Instrument parameters
In this application note, an iCAP MSX ICP-MS was operated
in conjunction with a Thermo Scientific™ iSC-65 Autosampler
for the accurate and reliable analysis of drinking and surface
water samples. A standard aqueous sample introduction system
was used, along with integrated argon gas dilution and typical
instrument parameters, as listed in Table 1. The Thermo Scientific™
Qtegra™ Intelligent Scientific Data Solution™ (ISDS) Software was
used to control the ICP-MS instrument and to generate, process,
and report analytical data. The method was set up as a template
in the software to ensure easy generation of data files in daily
analysis, where the entire workflow meets the quality control
requirements described in U.S. EPA Method 200.8.
Optimized instrument conditions were obtained using the
autotune routines included with the Qtegra ISDS Software to
ensure that analytical robustness and detection sensitivity are
achieved during analysis.
The ICP-MS was operated in standard mode, as the use of
traditional correction equations (Table 2) is mandated for the
mitigation of isobaric and polyatomic interferences when U.S.
EPA Method 200.8 is used for drinking water compliance
monitoring under the Safe Drinking Water Act and the National
Primary Drinking Water Regulations (NPDWR) 40 CFR Part 141.
The use of a collision/reaction cell (CRC) is not permitted. Internal
standardization was employed to monitor and compensate for
physical interferences, instrument drift, and signal suppression or
enhancement caused by the sample matrix.
Table 1. Instrument configuration and typical operating parameters Table 2. Summary of correction equations used for the target analytes
Parameter Value
Nebulizer iCAP MX Series ICP-MS nebulizer
Interface cones Ni – tipped sample and skimmer
Spray chamber Cyclonic quartz
Injector Quartz, 2.5 mm ID
Torch Quartz torch
Auxiliary flow (L·min-1) 0.8
Cool gas flow (L·min-1) 14
Nebulizer flow (L·min-1) 0.447
CRC conditions Not used as per U.S. EPA Method
200.8 v5.4 requirements
AGD setting, argon flow
(L·min-1)
AGD Level 5, 0.50
RF power (W) 1,550
Sampling depth (mm) 8
Number of replicates 3
Spray chamber temp. (°C) 2.7
Dwell time 0.05 s
Sweeps 5
Analytes Correction equation
As 3.127 [(77Se - 0.815 × 82Se)]
Cd 1.073 [(108Cd - 0.712 × 106Cd)]
Pb [
206Pb + 207Pb + 208Pb]
Mo [0.146 × 99Ru]
V 3.127 [(53Cr - 0.113 × 52Cr)]
Calibration standards
Calibration curves were generated for 21 analytes using six
calibration standards and a calibration blank. Multi-element
stock standards were prepared from single element standards of
each target analyte (1,000 mg·L-1, SPEX™ CertiPrep™, Metuchen,
NJ, USA). Two different stock solutions were prepared at a
concentration level of 2 mg·L-1 to accommodate analytes with
different concentrations and chemical compatibility. The stock
solutions were then diluted gravimetrically using 1% (v/v) nitric
acid as a diluent to result in the concentrations specified in
Table 3. An internal standard solution containing 20 µg∙L-1 of 6Li,
Sc, Y, Tb, and Bi was added on-line continuously during the
analysis. All solutions used for analysis consisted of 1% (v/v)
HNO3
in ultrapure water containing 100 µg∙L-1 Au as a stabilizer
for Hg.
2
Table 3. List of target analytes and their concentrations (µg·L-1) in calibration standards
Table 4. List of analytes and their MCLs in drinking waters allowed by NPDWR and NSDWR
Sample preparation
In total, five different water samples, collected locally, including
tap water (typical TDS level 100–300 mg·L-1), ground water
(typical TDS level 500–700 mg·L-1), and surface water (typical
TDS level up to 1,000 mg·L-1) were analyzed for the determination
of dissolved elements. The samples were filtered using 0.45 µm
pore size membrane filters followed by acidification using nitric
acid to adjust the nitric acid concentration of the samples to
approximately 1%. Multiple aliquots of these samples (equally
split between ground water, surface water, and tap water) were
then analyzed to assess accuracy and precision, performance
of duplicate measurements, and robustness over a 16-hour
analysis. A certified reference material (CRM) SLRS-5 (natural
river water) was included in the analysis as an additional
verification of accuracy.
Analytes STD 1 STD 2 STD 3 STD 4 STD 5 STD 6 STD 7
Hg 0 0.01 0.1 0.25 1 2 5
Al, Sb, As, Ba, Be, Cd, Cr, Co, Cu, Pb, Mn,
Mo, Ni, Se, Ag, Tl, Th, U, V, Zn 0 1 5 25 100 200 500
Please note that the concentration of selenium used in this study was 5 times lower compared to the requirements of EPA Method 200.8, Rev. 5.4
Results and discussion
Detection limits and linearity
The detection limits of the method must be below the standards
for inorganic contaminants in drinking waters set by the National
Primary Drinking Water Regulations (NSDWR) and the National
Secondary Drinking Water Regulations (NSDWR) (Table 4) with
the maximum contaminant level (MCL) and secondary maximum
contaminant level (SMCL) for each inorganic contaminant or
analyte in this study. Here, the method detection limits (MDLs) for
all analytes were calculated following the guidance provided in
U.S. EPA Method 200.8, Revision 5.4. Seven replicate aliquots of
fortified calibration blank (fortified at 2–5 times the concentration
level of the expected detection limits) were analyzed, and the
standard deviation of these measurements was multiplied by the
Student’s t value, which is 3.14 for seven replicate measurements.
The results are summarized in Table 5. The MDLs achieved
for all analytes were below the requirement of the regulations,
suggesting that the developed method enables sensitive
determination of all target analytes, meeting or exceeding the
requirements. The correlation coefficients (R2
) obtained for all
analytes were greater than 0.999, which suggests excellent
linear response for the established concentration range for each
analyte. The analytes, with their mass-to-charge ratio (m/z) and
calibration R2
are also summarized in Table 5.
MCL SMCL
Analyte MCL (mg∙L-1) Analyte MCL (mg∙L-1)
Sb 0.006 Al 0.05 to 0.2
As 0.010 Ag 0.1
Ba 2.0 Cu 1.0
Be 0.004 Mn 0.05
Cd 0.005 Zn 5
Cr 0.1
Pb 0.015
Hg 0.002
Se 0.05
Tl 0.002
3
Table 5. List of analytes, m/z, correlation coefficients, and MDLs
Analyte m/z R2 MDL (µg∙L-1)
Ag 107 0.9995 0.052
Al 27 0.9992 0.228
As 75 0.9995 0.046
Ba 137 0.9998 0.025
Be 9 0.9996 0.022
Cd 111 0.9998 0.022
Co 59 0.9994 0.010
Cr 52 0.9992 0.045
Cu 63 0.9995 0.061
Hg 202 0.9993 0.005
Mn 55 0.9990 0.026
Mo 98 >0.9999 0.018
Ni 60 >0.9999 0.037
Pb 206+207+208 0.9996 0.010
Sb 123 0.9994 0.020
Se 82 0.9998 0.193
Th 232 0.9991 0.046
Tl 205 0.9996 0.013
U 238 0.9993 0.024
V 51 0.9996 0.038
Zn 66 0.9995 0.122
Quality Control (QC) checks
Laboratory Reagent Blank (LRB) – LRB sample was prepared
from 1% (v/v) HNO3
in ultrapure water, which was treated similarly
as real samples by exposing it to the filtration through a 0.45 µm
pore diameter membrane filter. The LRB sample was analyzed
every 15 samples, and resulting concentrations of all analytes
were monitored. The concentrations of all analytes in subsequent
LRB samples were found to be within the acceptable range as
specified in U.S. EPA Method 200.8.
Laboratory Fortified Blank (LFB) – An aliquot of LRB was
spiked with stock solutions to yield the final concentration
of 75 µg∙L-1 for all analytes (except for Hg, which spiked at a
concentration level of 0.75 µg∙L-1). The recovery of each analyte
was calculated automatically within the Qtegra LabBook using
a recovery test available in the comprehensive QC toolkit. The
recovery values for all analytes were found to be within the range
of 85–115%, which suggests that the acceptance criteria were
met, and analysis could be continued.
Quality Control Sample (QCS) – QCS solution was prepared
by spiking an aliquot of the LRB using independent analyte stock
solutions (different from the stock solutions used for preparation
of calibration standards) to yield analyte concentrations at
50 µg∙L-1 level (except for Hg, which was spiked at 0.5 µg∙L-1
concentration level). The QCS was used to confirm the initial
validity of calibration standards as well as to confirm the ongoing
instrument performance during the measurement of more than
16 hours. The QCS was analyzed every 10 samples, with the
percent accuracy for all analytes in each QCS found to be well
within the acceptable range of ±10% (equivalent to 90–110%) of
the true concentration.
Accuracy
Laboratory Fortified Matrix (LFM) – To assess and
demonstrate method accuracy and precision, one of the water
samples was spiked in duplicate at a concentration level of
50 µg∙L-1 for all analytes (except for Hg, which was spiked at a
concentration level of 0.5 µg∙L-1). The percent recovery of each
analyte was calculated automatically within Qtegra ISDS Software
based on the measured concentration for the unspiked and
spiked replicates of the LFM. The average percent recovery for
all analytes was found to be within the range of 85–115%, against
the acceptable range of 70–130%, with relative percent difference
of less than 5% between duplicate spike measurements. Table 6
lists the analytes and their average percent recovery calculated
from duplicate measurements of spiked water sample.
Analysis of Certified Reference Material (CRM) and
observed accuracy – To further demonstrate method accuracy,
a certified reference material (SLRS-5, natural river water) was
analyzed three times during a long-term analysis of more than
16 hours. The percent accuracy of each analyte was calculated
based on the concentration data obtained during the analysis
and the certified values given for each analyte in the SLRS-5
CRM. Table 7 summarizes the list of analytes, their certified
concentration values, and calculated percent accuracy. The
percent accuracy values given are the average calculated from
three different measurements of SLRS-5 CRM.
4
Table 6. Average percent recovery calculated from the duplicate measurement of spiked water sample (spike levels 0.5 µg∙L-1 for Hg and
50 µg∙L-1 for other analytes)
Table 7. List of analytes, certified concentrations and accuracy (as percent) of the certified reference material (CRM) SLRS-5
Analyte Concentration in unspiked
water sample (µg∙L-1)
Measured spike
concentration (µg∙L-1) Spike recovery (%), n=2
Ag 2.4 54.1 108.2
Al 15.5 48.9 97.8
As 0.015 52.4 104.9
Ba 14.3 51.1 102.3
Be 0.036 52.5 105.1
Cd 0.032 51.0 102.1
Co 0.087 51.0 102.0
Cr 0.084 51.0 102.0
Cu 89.9 52.2 104.4
Fe 91.3 52.0 104.0
Hg 0.019 0.52 104.9
Mn 0.011 51.9 103.9
Mo 0.312 52.3 104.6
Ni 5.4 46.8 93.6
Pb 0.304 54.0 108.0
Sb 0.024 54.8 109.6
Se 0.122 49.5 99.1
Th 0.095 55.2 110.3
Tl 0.158 54.0 107.9
U 0.055 55.1 110.3
V <DL 51.6 103.3
Zn 79.3 49.9 99.7
Analyte Certified concentration (µg∙L-1) Accuracy (%), n=3
Al 49.5 ± 5.0 95.2 ± 5
As 0.413 ± 0.039 94.2 ± 7
Ba 14.0 ± 0.5 112.1 ± 3
Be 0.005* 100.0 ± 3
Co 0.05* 106.8 ± 3
Cr 0.208 ± 0.023 93.8 ± 6
Cu 17.4 ± 1.3 94.4 ± 2
Fe 91.2 ± 5.8 105.1 ± 6
Mn 4.33 ± 0.18 104.2 ± 4
Mo 0.27 ± 0.04 103.4 ± 5
Ni 0.476 ± 0.064 91.6 ± 1
Pb 0.081 ± 0.006 97.3 ± 2
Sb 0.3* 86.2 ± 4
U 0.093 ± 0.006 93.0 ± 7
V 0.317 ± 0.033 101.3 ± 2
Zn 0.845 ± 0.095 97.4 ± 3
*Information values, as per CRM certificate of analysis
5
Method robustness
A robust instrument and optimized method are key requirements
when analyzing varying sample matrices within an analytical run.
Instrument drift and physical interferences due to the sample
matrices can cause signal enhancement or suppression and
must be corrected for. In addition, U.S. EPA Method 200.8
includes specific guidelines and requirements, such as internal
standardization, to monitor and correct for instrument drift and
physical interferences. An internal standard must be added to all
standards and samples in the run sequence and must not deviate
outside of the acceptable range of 60–125% compared to the
response observed in the calibration blank.
As previously mentioned, an internal standard solution containing
20 µg∙L-1 of 6Li, Sc, Y, Tb, and Bi was added online during the
analysis. Figure 1 shows a plot of the internal standards recovery
against the calibration blank throughout the 16-hour analysis. As
shown, all internal standards recoveries were within the range of
80–112%, well within the acceptance criteria of U.S. EPA Method
200.8. These results demonstrate the robustness of the method
and instrument setup that included online sample dilution.
Conclusions
The quality of analytical data obtained during this study indicates
that the iCAP MSX ICP-MS, equipped with a built-in AGD system
for controlled and automatic dilution of the sample aerosol, is
a powerful solution for the analysis of varying sample matrices
within a single run sequence. Some of the important outcomes of
this study are highlighted here.
Figure 1. Internal standard recovery plot from a Qtegra LabBook, highlighting the consistent recovery of internal
standards within 80–112% throughout the 16-hour continuous analysis
• The MDLs achieved comfortably meet the requirements of
the method and were below the regulations for inorganic
contaminants in drinking water. This indicates that the
instrument setup, with optimized sample dilution, provides the
sensitivity and robustness for the analysis of a variety of water
samples in a single, extended analysis.
• The accuracy and precision obtained from the analysis
of the LFM and SLRS-5 CRM indicate that the developed
method and instrument setup were optimized for the
variety of samples analyzed. Furthermore, the ease of
instrument tuning, operation, and LabBook set up through
the Qtegra ISDS Software allows routine analysis of varying
environmental samples with minimal effort while improving
productivity.
• The accurate results obtained from the analyses of the QCS
samples demonstrate on-going instrument performance
and calibration over the extended analysis of varying sample
matrices.
• The internal standard recoveries of 80–112% observed
during the 16-hour analysis demonstrate robustness of the
instrument and that the dilution applied to each sample was
helpful in minimizing the effects of physical interferences.
• The QC toolkit included within the Qtegra ISDS Software
enables easy set up and compliance with the comprehensive
QC protocol of U.S. EPA Method 200.8. Customizable report
templates are available to provide all data required for audit
and regulatory purposes. The use of the flags and limits
features within the software helps highlight and call out data
falling outside the acceptable concentration ranges and
provides easy visualization and review of data.
6
General Laboratory Equipment – Not For Diagnostic Procedures. © 2024 Thermo Fisher Scientific Inc. All rights reserved. All
trademarks are the property of Thermo Fisher Scientific and its subsidiaries unless otherwise specified. SPEX CertiPrep is a trademark
of SPEX CertiPrep, Inc. This information is presented as an example of the capabilities of Thermo Fisher Scientific products. It is not
intended to encourage use of these products in any manner that might infringe the intellectual property rights of others. Specifications,
terms and pricing are subject to change. Not all products are available in all countries. Please consult your local sales representative for
details. AN002911 0424
Learn more at thermofisher.com/icp-ms
References
1. U.S. EPA Method 200.8. https://www.epa.gov/sites/default/files/2015-08/documents/
method_200-8_rev_5-4_1994.pdf
2. National Primary Drinking Water Regulations. https://www.epa.gov/
ground-water-and-drinking-water/national-primary-drinking-water-regulations
3. National Primary Drinking Water Regulations. https://www.epa.gov/sdwa/secondarydrinking-water-standards-guidance-nuisance-chemicals#what-are-secondary
Brought to you by
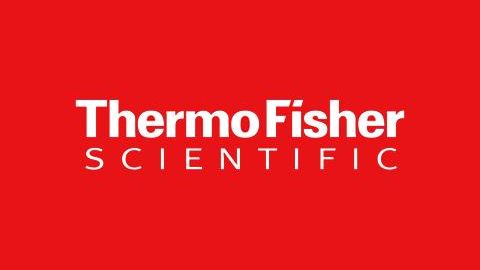
Download the Application Note for FREE Now!
Information you provide will be shared with the sponsors for this content. Technology Networks or its sponsors may contact you to offer you content or products based on your interest in this topic. You may opt-out at any time.
Experiencing issues viewing the form? Click here to access an alternate version