Testing for PFOS, PFOA and Other Related PFAS Compounds
Discover the chemical properties, health effects and regulatory limits of PFAS compounds and how to detect them.
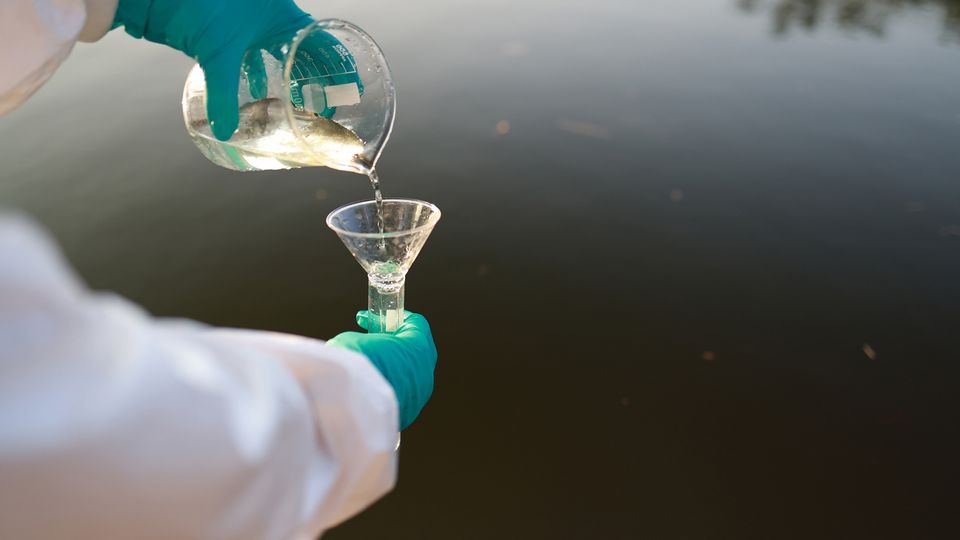
Complete the form below to unlock access to ALL audio articles.
Per- and polyfluoroalkyl substances (PFAS) are synthetic chemicals widely used in consumer and industrial products for their durability and resistance to heat, water and stains. However, their persistence in the environment and potential health risks have raised concerns, leading to increased regulatory scrutiny.
This article provides an overview of PFAS, including their chemical properties, health effects and regulatory limits. It also explores PFAS detection methods, with a focus on EPA Method 1633, a key analytical tool. Finally, we compare targeted and non-targeted analyses, highlighting their applications in PFAS monitoring and research. Understanding these aspects is essential for addressing the challenges posed by these "forever chemicals."
What are PFAS?
- What is PFOS (perfluorooctanesulfonic acid)?
- What is a PFAA (perfluoroalkyl acid)?
- What is PFOA (perfluorooctanoic acid)?
- What is PFBS (perfluorobutanesulfonic acid)?
Other related "forever chemicals"
Advisory limits for PFAS compounds
What are PFAS?
PFAS refers to both "perfluoroalkyl substances" and "polyfluoroalkyl substances," a large group of human-made chemicals that contain a high percentage of fluorine atoms.1 What makes them unique is the strength of the carbon-fluorine bond, one of the strongest in chemistry. This bond makes PFAS extremely stable, so manufacturers have widely used them in non-stick cookware, waterproof clothing, stain-resistant carpets, firefighting foams and even personal care products like makeup and shampoo.2 These chemicals were first developed for industrial use in the 1940s, and industries quickly realized that incorporating them into their products conveyed unique advantages.
However, the high stability of PFAS has a significant downside: PFAS do not break down easily. They degrade so slowly that if you dispose of a PFAS-containing product today, traces of it could still be found in the environment centuries from now. Because of this extreme persistence, PFAS have earned the nickname "forever chemicals."3
PFAS don’t just sit in landfills – they spread. These chemicals leach into water and soil, contaminating ecosystems and accumulating in living organisms. Over time, PFAS levels build up in plants, animals and humans – a process known as bioaccumulation. Worse, as PFAS move up the food chain, they become more concentrated in top predators. This effect, called biomagnification, means that those higher on the food chain, like humans, are exposed to higher PFAS levels over time.4
Biomagnification is particularly concerning because PFAS exposure has been linked to serious health problems, including an increased risk of cancer,5 thyroid disease,6 infertility,7 immune dysfunction8 and liver damage.9 Once PFAS enter the environment or the human body, they don’t just disappear – they build up, potentially leading to serious health problems later on.
PFAS structure
PFAS are a class of compounds with a carbon-based backbone and a lot of fluorine (F) atoms.
Most organic compounds consist of carbon (C) and hydrogen (H) atoms connected by single bonds, forming alkyl substances. These carbon-hydrogen (C-H) bonds are relatively strong but can still be broken down through natural processes, such as microbial degradation or oxidation. In perfluoroalkyl substances, every one of the hydrogen atoms has been replaced with a fluorine atom, which means that the molecules have only C-C and C-F bonds. In polyfluoroalkyl substances, by contrast, only some of the hydrogen atoms have been replaced with fluorine atoms, meaning there are C-C, C-F and C-H bonds, as shown in Figure 1.
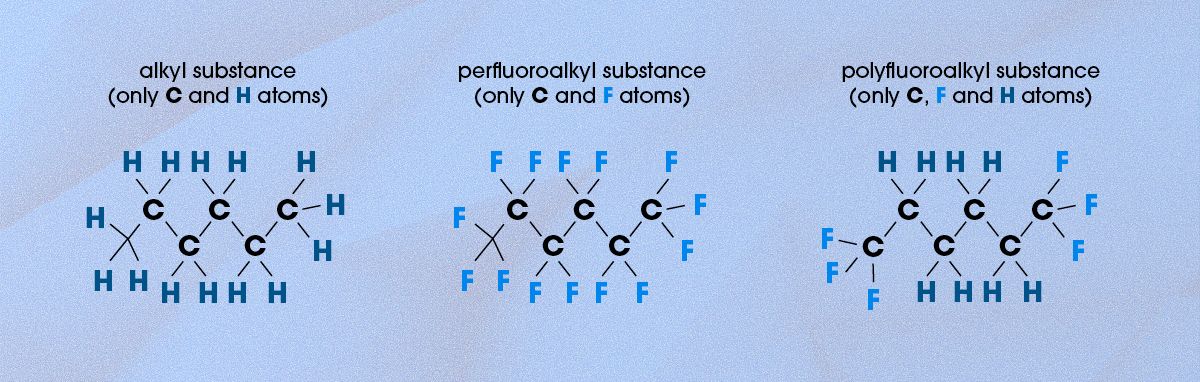
Figure 1. Examples of the structures of alkyl, perfluoroalkyl and polyfluoroalkyl substances. Credit: Technology Networks.
The presence of fluorine is crucial because C-F bonds are among the strongest chemical bonds. This makes PFAS highly resistant to heat, water and chemical degradation, explaining their persistence in the environment and biological systems.10 Unfortunately, this same durability makes them difficult to remove once they contaminate ecosystems or enter the human body.
What is PFOS (perfluorooctanesulfonic acid)?
PFOS stands for perfluorooctanesulfonic acid, and its chemical structure is shown in Figure 2.11 Structurally, PFOS is similar to the perfluoroalkyl substances shown in Figure 1 but with an added SO₃H group, known as a sulfonic acid, on one end of the molecule. Because sulfonic acid is a strong acid, it readily donates a proton (H⁺), meaning that PFOS is almost always found in its anionic (negatively charged) sulfonate form (Figure 2).
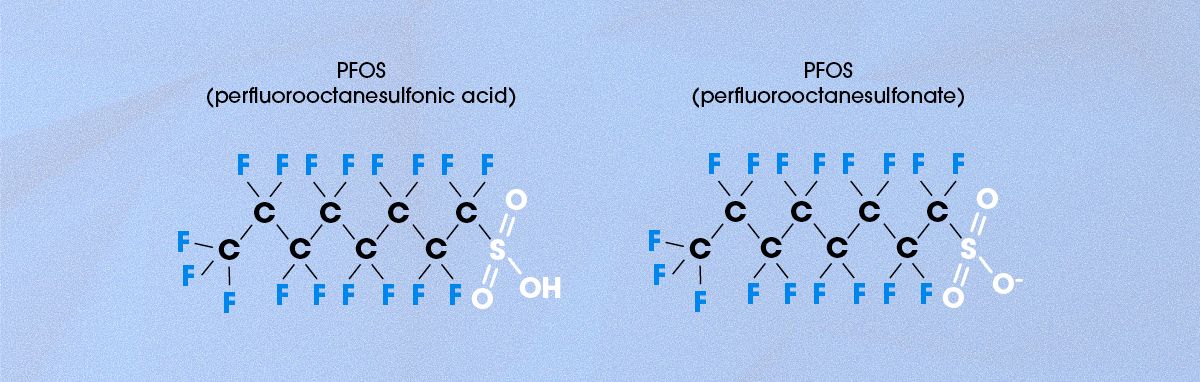
Figure 2. Chemical structure of PFOS in its neutral and anionic form. Credit: Technology Networks.
PFOS is a type of PFAS, despite its sulfonic acid group, and has been one of the most widely used PFAS chemicals. It has been used in numerous commercial products, including stain-resistance fabric coatings (i.e., Scotchgard), firefighting foams, waterproof makeup and cosmetics and cleaning and polishing products. PFOS is particularly well-suited for these applications because it combines the exceptional stability of all PFAS with the polar sulfonic acid group, which enhances its water solubility and gives it surfactant-like properties. These features make PFOS highly effective at repelling water, grease and stains while ensuring long-lasting performance.12
What is a PFAA (perfluoroalkyl acid)?
PFAAs are molecules with a fully fluorinated carbon backbone, which have either a carboxylic acid or sulfonic acid functional group at one end of the structure (Figure 3). This means that they have all the advantages of PFAS molecules (high stability) and can often be dissolved in water (due to the polar acid group).
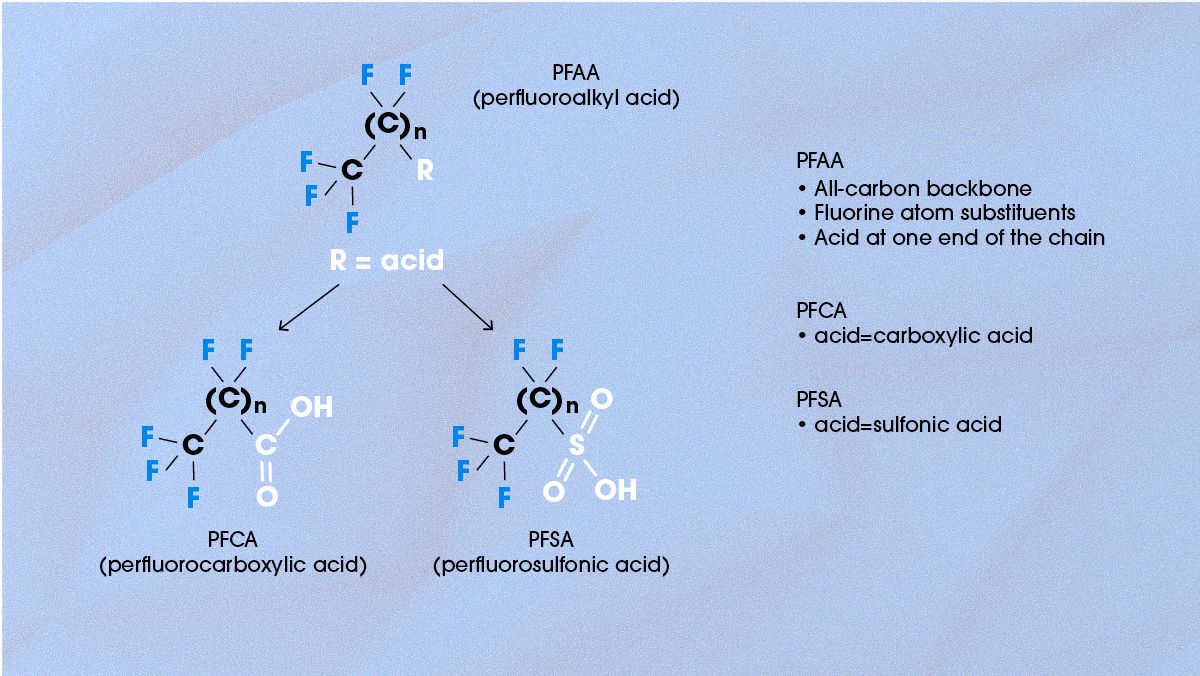
Figure 3. The chemical structure of PFAA and the difference between PFCA and PFSA. Credit: Technology Networks.
What is PFOA (perfluorooctanoic acid)?
PFOA, or perfluorooctanoic acid, is another widely recognized PFAS compound.13 While structurally similar to PFOS, PFOA differs in one key way: instead of a sulfonic acid (-SO₃H) group, it contains a carboxylic acid (-COOH) group at one end of the molecule (Figure 4). Because of this structure, PFOA is amphiphilic, meaning it has a polar end (the carboxylic acid group) and a non-polar end (the perfluoroalkyl chain). The structure of PFOA explains its unique properties, which include:
- High stability due to the strength of its C-F bonds
- Moderate aqueous solubility due to the carboxylic acid group
- High solubility in fluorinated solvents due to the perfluoroalkyl chain
- Surfactant-like behavior, which means that it adsorbs at interfaces and lowers the surface tension
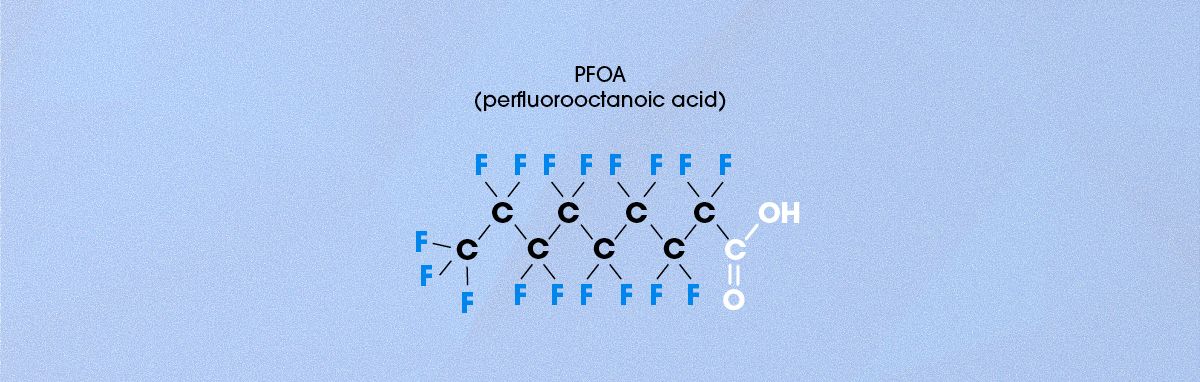
Figure 4. Chemical structure of PFOA. Credit: Technology Networks.
Because PFOS and PFOA have similar properties, they were used in the same products, such as floor polishes, car waxes and semiconductor manufacturing. However, PFOS was more common in stain-resistant textiles and firefighting foams, while PFOA was more commonly used in non-stick coatings and food packaging.
What is PFBS (perfluorobutanesulfonic acid)?
Another important PFAS chemical is PFBS, or perfluorobutanesulfonic acid (Figure 5). PFBS is structurally similar to PFOS, except its perfluoroalkyl chain is much shorter (four carbons in PFBS vs eight carbons in PFOS). Due to this structural difference, PFBS is more water-soluble, making it less likely to bioaccumulate in organisms or adhere to soil and sediment within an ecosystem. It also degrades more readily in water than PFOS, with the estimated half-life of PFBS in water somewhere between 80 and 100 days (compared to 41 years for PFOS). However, the high aqueous solubility of PFBS means that it can easily contaminate aqueous environments, including the drinking water supply, and spread over a large geographic area.14
When PFOS was phased out and banned in the early 2000s, manufacturers turned to PFBS as a safer alternative.15 Since then, PFBS has been widely used in many of the commercial products that previously used PFOS, including stain-resistant and water-resistant fabric coatings, grease-resistant food packaging and parchment paper, firefighting foams and waterproof cosmetics and hairsprays. However, as the use of PFBS has increased, concerns about its potential health and environmental risks have grown. Many regulators, consumers and manufacturers are currently reviewing the widespread use of PFBS, which suggests that its usage may be curtailed in the near future.
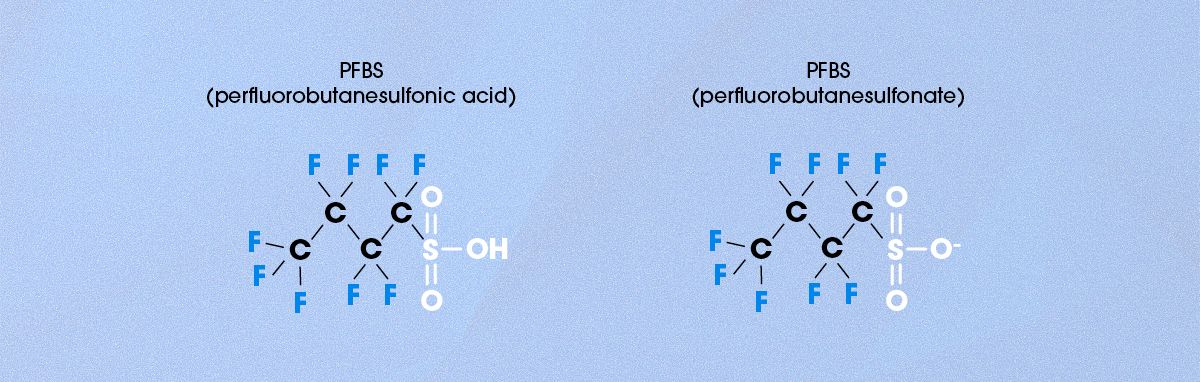
Figure 5. Chemical structure of PFBS in its neutral and anionic form. Credit: Technology Networks.
Other related “forever chemicals”
There are currently over 12,000 known PFAS chemicals. While we have covered some of the most well-known, several others deserve attention due to their widespread use and potential health and environmental risks (Figure 6).
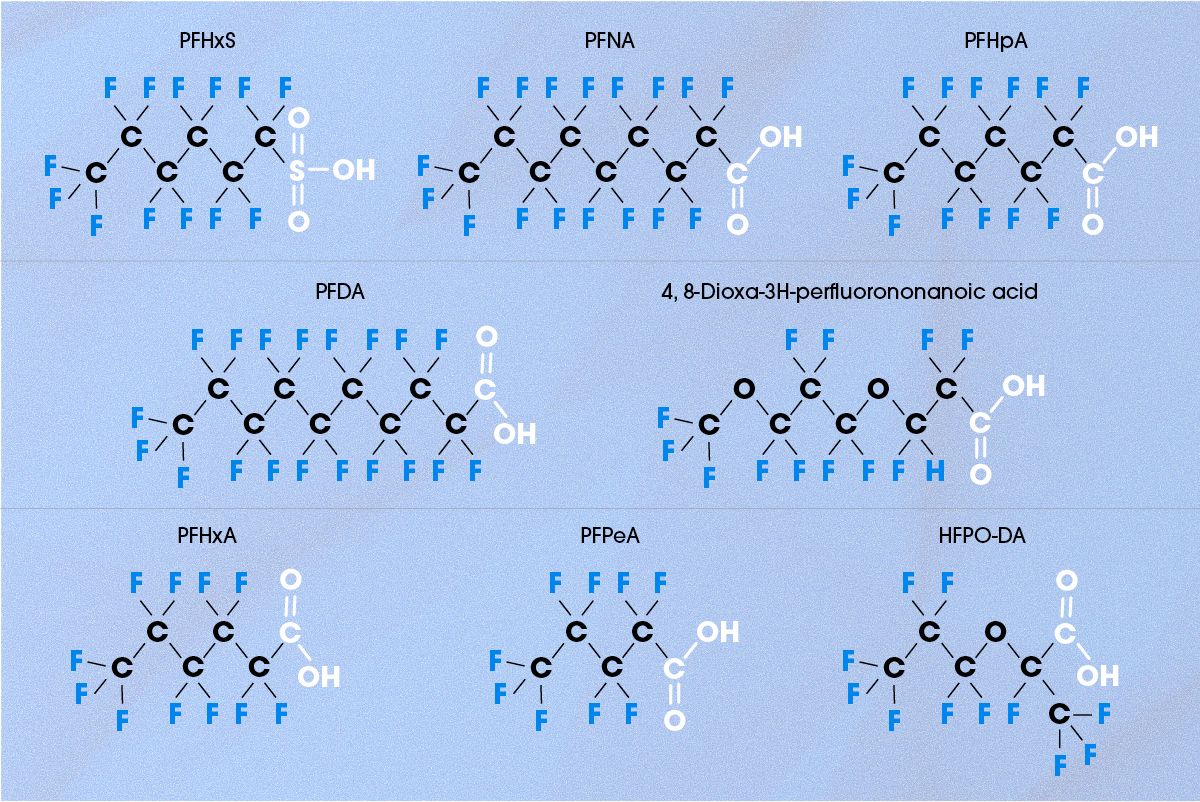
Figure 6. Chemical structures of other related PFAS chemicals. Credit: Technology Networks.
Perfluorohexanesulfonic acid (PFHxS)
PFHxS is a perfluorosulfonic acid (PFSA) with a six-carbon chain.16 It has properties similar to PFOS and has been used as a replacement in firefighting foams, textiles and coatings. However, PFHxS exposure has been shown to increase the rates of thyroid disease,17 liver dysfunction18 and developmental delays,19 and it is also highly environmentally persistent. Its usage is highly regulated in several countries, although its past widespread usage and environmental persistence means that significant PFHxS contamination remains.
Perfluorononanoic acid (PFNA)
PFNA is a perfluorocarboxylic acid (PFCA) with a nine-carbon chain, structurally similar to PFOA.20 However, its slightly larger size means it is more likely to bioaccumulate and persist in the environment. PFNA is commonly used in non-stick cookware, grease-resistant food packaging and specialty electronics as a PFOA alternative. Moreover, unlike PFOA, PFNA exposure has not been directly linked to cancer, although studies have shown that it may be toxic to multiple body systems.21,22
Perfluoroheptanoic acid (PFHpA)
PFHpA is another PFCA with a seven-carbon chain.23 It is highly stable and environmentally persistent, much like its longer-chain counterparts, though it is more water-soluble, allowing it to remain longer in the water supply. This increased solubility makes it less likely to bioaccumulate in humans and wildlife, though it still poses risks due to its resistance to degradation.
Research on the health effects of PFHpA exposure is limited, but early studies suggest potential liver24 and immune system25 effects. Because of this lack of conclusive data, PFHpA has not yet been banned, but regulators are beginning to examine its risks more closely.
Perfluorodecanoic acid (PFDA)
PFDA is a ten-carbon PFCA, with limited water solubility but a high likelihood of bioaccumulation.26 It shares many chemical properties with PFNA and PFOA, meaning it is highly stable and long-lasting in the environment. PFDA has been used in industrial coatings and specialty textiles but concerns over its reproductive toxicity and liver damage have led to increased scrutiny. While PFDA remains unregulated in the United States (US), it is highly restricted in the European Union (EU) due to its persistence and potential health risks.
PFHxA (perfluorohexanoic acid)
PFHxA is a six-carbon PFCA that is more water-soluble and more easily degraded than longer-chain PFCAs. This increased solubility allows it to contaminate groundwater and drinking water supplies, though it is less likely to bioaccumulate in living organisms. PFHxA has been widely used in non-stick cookware, baking sheets and water-resistant textiles, often replacing PFOA. While its toxicity has not been as well studied as other PFAS, some research suggests potential liver and immune system effects.27,28
PFPeA (perfluoropentanoic acid)
PFPeA is a five-carbon PFCA that has not been as heavily regulated as its longer-chain counterparts. Because its shorter chain length makes it less environmentally persistent, it has a reduced likelihood of bioaccumulation and biomagnification in humans and wildlife. Notably, its long-term health effects have not been well-studied, and it continues to be widely used in industrial applications, specialty coating and fluoropolymer processing.29
GenX (hexafluoropropylene oxide dimer acid (HFPO-DA))
GenX is a perfluoroalkyl ether carboxylic acid (PFECA) that was developed by DuPont in 2010 as a replacement for PFOA. While it was marketed as a safer alternative, it has many of the chemical properties of PFOA, including high water solubility, allowing it to spread rapidly in groundwater.30 GenX has been used in manufacturing Teflon coatings and other industrial applications, although emerging research indicates that exposure to GenX can cause significant harm. Although GenX is not currently banned in the US or Europe, regulatory agencies have begun regulating its concentration in drinking water.
4,8-Dioxa-3H-perfluorononanoic acid
4,8-Dioxa-3H-perfluorononanoic acid is another PFECA used primarily in fluoropolymer production. While it is chemically similar to HFPO-DA (GenX), it is less water-soluble and less likely to contaminate groundwater and aquatic ecosystems.31 Toxicity studies on 4,8-dioxa-3H-perfluorononanoic acid remain limited, though early data suggests potential health risks similar to GenX. Because current regulations do not limit the use of 4-8-dioxa-3H-perfluorononanoic acid, it continues to be used in industry, mostly in fluoropolymer production processes.
PFAS health effects
While the health effects of all 12,000+ PFAS chemicals have not been fully studied, extensive research has linked certain PFAS compounds to serious health risks. Below are five of the most well-documented adverse health effects associated with PFAS exposure.
1. Cancer
Exposure to certain PFAS chemicals has been linked to an increased risk of certain cancers. More specifically, a 2020 study found that individuals with high concentrations of PFOA in their blood had more than twice the risk of developing kidney cancer compared to those with lower levels.32 Similarly, a 2023 study on active-duty US Air Force servicemen found that those with high PFOS levels were significantly more likely to develop testicular germ cell tumors.33 However, research has not found a clear link between PFAS exposure and aggressive prostate cancer,34 and studies on the connection between breast cancer risk and PFAS exposure have yielded inconclusive results.35
2. Liver damage
PFAS exposure has been strongly associated with liver disease. In animal studies, rats with higher PFOA, PFOS and PFNA concentrations in their blood exhibited elevated levels of alanine aminotransferase (ALT), a key marker of liver dysfunction.36 This trend was also observed in human subjects, where high PFOA levels correlated with increased ALT levels, indicating potential liver damage.37 A separate study published in 2022 found that individuals with elevated PFOS levels in their blood serum had an increased risk of developing liver cancer.38
3. Thyroid dysfunction
Studies suggest that PFAS exposure can disrupt thyroid function, with both cancerous and non-cancerous effects. Researchers from Mount Sinai Hospital found that individuals with the highest PFOS concentrations in their blood had a 56% higher risk of developing thyroid cancer compared to those with lower levels.39 Even in non-cancer cases, PFAS exposure appears to alter thyroid hormone regulation. For example, one study showed that people with high PFOA levels had reduced T4 hormone function, while elevated PFOS and PFNA levels were linked to reduced thyroid-stimulating hormone activity, which can affect metabolism and overall thyroid health.40
4. Immune system suppression
PFAS exposure has been shown to weaken immune system function. In laboratory studies, mice exposed to PFOA exhibited changes in the number and type of B cells (a key component of the immune system) and altered rates of B-cell activation.41 Moreover, molecular biology experiments have demonstrated that PFOS activates the AIM2 inflammasome, a cellular sensor that triggers strong inflammatory responses. This process leads to the self-destruction of immune cells and an overall weakening of the immune system over time, reducing the body’s ability to fight infections.42
5. Reproductive and developmental issues
Developmental toxicity from PFAS exposure has been documented in multiple animal studies. In a 2024 study, researchers found that zebrafish embryos exposed to PFOS experienced significant developmental toxicity, with some less-studied PFAS chemicals proving even more toxic.43 Interestingly, researchers found no clear correlation between PFOS and PFOA blood serum levels and sperm volume, concentration or motility.44 A third study found that mothers with higher levels of PFOA in their blood had children who scored lower on developmental milestone exams, suggesting that prenatal exposure may negatively impact early childhood development.45
Advisory limits for PFAS chemicals
The advisory limits for PFAS set out by a regulator depend on several factors:
- The identity of the PFAS compound, with longer-chain perfluoroalkyl compounds such as PFOA and PFAS generally more restricted compared to their shorter-chain analogs.
- The regulatory body, with the EU generally imposing more stringent restrictions on PFAS usage and exposure limits compared to the US.
- The environmental medium, with drinking water regulations generally far stricter than the limits set for air or soil contamination.
The current regulatory limits for PFAS in drinking water, as set by the US Environmental Protection Agency (EPA)46 and the EU,47 are summarized in Table 1.
Table 1. Maximum allowable PFAS concentrations in drinking water (US vs EU).
Compound | US (EPA) limit | EU limit |
PFOS | 4 parts per trillion (ppt) | 0.1 micrograms per liter (μg/L) for individual PFAS, 0.5 μg/L for total PFAS |
PFOA | 4 ppt | |
PFBS | 2,000 ppt | |
PFBA | Not determined | |
HFPO-DA | 10 ppt |
Of note, the EU regulations do not distinguish between different PFAS chemicals, even though it is generally accepted that longer-chain PFAS are more toxic than their shorter-chain counterparts. Additionally, the EU enforces a cumulative PFAS concentration limit in drinking water, a precaution not currently addressed in the US regulatory framework.
Methods for PFAS analysis
Given the environmental persistence and potential dangers of PFAS chemicals, it is clear that we need rapid, sensitive and selective methods to detect PFAS in complex environments, including air, soil and water. The currently utilized methods for PFAS detection can be divided into two broad categories:
- Instrument-based methods, which require sophisticated laboratory instrumentation and are typically conducted in controlled laboratory settings.
- Non-instrument-based methods, which involve portable detection systems that can be used for on-site field testing without the need for expensive equipment or a laboratory environment.
The EPA’s regulatory-approved methods for PFAS detection are instrument-based and vary significantly depending on the sample type being analyzed. A summary of these regulatory-approved methods is shown in Table 2.
Table 2. A summary of the EPA's regulatory-approved methods for PFAS detection in various sample types.
Sample Type | EPA-Approved Method(s) | Key Features of Method(s) |
Drinking water | EPA Method 537.148 | Solid-phase extraction (SPE), liquid chromatography-tandem mass spectrometry (LC-MS/MS) |
EPA Method 53349 | Isotope dilution anion exchange SPE, LC-MS/MS | |
Other water (groundwater, surface water, wastewater) | EPA Method 832750 | External standard calibration; LC-MS/MS |
Soil, sediment, biosolids, landfill material, fish tissues | EPA Method 163351 | Analysis in aqueous, solid, biosolids and tissue samples by LC-MS/MS |
Air emissions | Other test method (OTM) 4552 | Measures volatile and semi-bound PFAS using LC-MS/MS |
Food | FDA method53 | LC-MS/MS |
While non-instrument-based methods for PFAS detection are generally not regulatory-approved, they provide significant advantages for field testing and rapid screening. These methods allow researchers, environmental scientists and first responders to detect PFAS without needing laboratory infrastructure. This is a highly active research area, with new detection techniques emerging in fields such as:
- Colorimetric detection (visual color changes in response to PFAS)54
- Electrochemical sensors (detecting electrical changes upon PFAS interaction)55
- Fluorescence-based detection (fluorogenic molecules that respond to PFAS presence)56
Although these methods are not replacements for regulatory-approved testing, they serve as first-line screening tools, helping to identify potentially contaminated sites that require further investigation with EPA-approved laboratory methods.
EPA method 1633 for PFAS analysis
Among the various EPA-approved methods, EPA Method 1633 stands out for several reasons:
(1) Broad sample scope
Unlike previous methods that focus on a single matrix type, EPA Method 1633 includes treatment protocols for various sample types, including contaminated water, soil, sediment, biosolids and fish tissue samples.
(2) Large number of target analytes
This method detects 40 specific PFAS compounds, significantly more than EPA Method 537.1 or EPA Method 533. This list includes widely known contaminants such as PFOA and PFOS, perfluoroalkyl sulfonamides, perfluoroether carboxylic acids and perfluoroalkyl sulfonamidoacetic acids.
(3) Extremely high sensitivity
In water samples, EPA Method 1633 can detect PFAS at extremely low concentrations, often below 1 nanogram per liter (ng/L). This sensitivity is achieved through the use of isotopically-labeled standards (which provide a sensitive internal standard for quantification) and mass spectrometry-based detection.
(4) Developed in collaboration with multiple agencies
Unlike earlier EPA regulatory methods, EPA Method 1633 was developed with the US Department of Defense. This ensures standardized protocols that meet environmental monitoring needs and defense-related contamination assessments.
EPA Method 1633 begins with sample preparation, where a known amount of labeled PFAS compounds is used as a reference. SPE is used to isolate PFAS from other substances present in the sample.
Once prepared, the sample is analyzed using LC-MS/MS. This technique separates, identifies and measures PFAS based on their unique chemical signatures. The results are compared to the labeled reference compounds, ensuring reliable and precise detection, even at very low levels.
Targeted vs non-targeted analysis
There are two main approaches to detecting PFAS in the environment: targeted analysis and non-targeted analysis. Each serves a different purpose in identifying contamination.
Targeted analysis looks for specific, known PFAS chemicals by comparing a sample to a reference standard. This method is precise, widely used in regulatory testing and performed with advanced instruments like LC-MS/MS. However, it can only detect PFAS that scientists already know to look for.
Non-targeted analysis, by contrast, scans for any fluorine-containing compounds, including previously unidentified PFAS. Instead of searching for specific chemicals, this approach screens for molecular patterns using high-resolution mass spectrometry. While this method helps scientists discover new PFAS, it is less precise for measuring exact amounts of contamination.
Deciding between targeted and non-targeted PFAS analysis depends on your application. Targeted analysis is useful when measuring specific PFAS compounds, for example, to ensure compliance with regulation, but it cannot detect unknown or emerging compounds. Non-targeted analysis is more useful in complex environmental contamination scenarios, where the scope of contamination and types of PFAS present have not yet been determined. Still, it is harder to obtain quantitative information from non-targeted analysis and data interpretation is generally complicated.
1. Sunderland EM, Hu XC, Dassuncao C, Tokranov AK, Wagner CC, Allen JG. A review of the pathways of human exposure to poly- and perfluoroalkyl substances (PFASs) and present understanding of health effects. J Expo Sci Environ Epidemiol. 2019;29(2):131-147. doi: 10.1038/s41370-018-0094-1.
2. Tansel B. PFAS use in electronic products and exposure risks during handling and processing of e-waste: A review. J Environ Manage. 2022;316:115291. doi: 10.1016/j.jenvman.2022.115291.
3. Wee SY, Aris AZ. Revisiting the “forever chemicals”: PFOA and PFOS exposure in drinking water. npj Clean Water. 2023;6(1):57. doi: 10.1038/s41545-023-00274-6.
4. Domingo JL, Nadal M. Human exposure to per- and polyfluoroalkyl substances (PFAS) through drinking water: A review of the recent scientific literature. Environ Res. 2019;177:108648. doi: 10.1016/j.envres.2019.108648.
5. Steenland K, Winquist A. PFAS and cancer, a scoping review of the epidemiologic evidence. Environ Res. 2021;194:110690. doi: 10.1016/j.envres.2020.110690.
6. Coperchini F, Croce L, Ricci G, et al. Thyroid disrupting effects of old and new generation PFAS. Front Endocrinol. 2021;11:612320. doi: 10.3389/fendo.2020.612320.
7. Wang W, Hong X, Zhao F, Wu J, Wang B. The effects of perfluoroalkyl and polyfluoroalkyl substances on female fertility: A systematic review and meta-analysis. Environ Res. 2023;216(Pt 3):114718. doi: 10.1016/j.envres.2022.114718.
8. DeWitt JC, Blossom SJ, Schaider LA. Exposure to per-fluoroalkyl and polyfluoroalkyl substances leads to immunotoxicity: epidemiological and toxicological evidence. J Expo Sci Environ Epidemiol. 2019;29(2):148-156. doi: 10.1038/s41370-018-0097-y.
9. Zhao L, Zhang X, Ducatman A, Zhang X. Associations between per- and polyfluoroalkyl substances and adolescent non-alcoholic fatty liver disease. J Hepatol. 2023;79(5):e208-e210. doi: 10.1016/j.jhep.2023.07.008.
10. Antonopoulou M, Spyrou A, Tzamaria A, Efthimiou I, Triantafyllidis V. Current state of knowledge of environmental occurrence, toxic effects, and advanced treatment of PFOS and PFOA. Sci Total Environ. 2024;913:169332. doi: 10.1016/j.scitotenv.2023.169332.
11. Lim TC, Wang B, Huang J, Deng S, Yu G. Emission inventory for PFOS in China: Review of past methodologies and suggestions. Sci World J. 2011;11:1963-1980. doi: 10.1100/2011/868156.
12. Sungur Ş. Dietary exposure to perfluorooctanoic acid (PFOA) and perfluorooctane sulfonic acid (PFOS): a review of recent literature. Toxin Rev. 2018;37(2):106-116. doi: 10.1080/15569543.2017.1346685.
13. Prevedouros K, Cousins IT, Buck RC, Korzeniowski SH. Sources, fate and transport of perfluorocarboxylates. Environ Sci Technol. 2006;40(1):32-44. doi: 10.1021/es0512475.
14. Ivantsova E, Lu A, Martyniuk CJ. Occurrence and toxicity mechanisms of perfluorobutanoic acid (PFBA) and perfluorobutane sulfonic acid (PFBS) in fish. Chemosphere. 2024;349:140815. doi: 10.1016/j.chemosphere.2023.140815.
15. Wang Z, Cousins IT, Scheringer M, Hungerbühler K. Fluorinated alternatives to long-chain perfluoroalkyl carboxylic acids (PFCAs), perfluoroalkane sulfonic acids (PFSAs) and their potential precursors. Environ Int. 2013;60:242-248. doi: 10.1016/j.envint.2013.08.021.
16. Ali JM, Roberts SM, Gordon DS, Stuchal LD. Derivation of a chronic reference dose for perfluorohexane sulfonate (PFHxS) for reproductive toxicity in mice. Regul Toxicol Pharmacol. 2019;108:104452. doi: 10.1016/j.yrtph.2019.104452.
17. Long M, Ghisari M, Bonefeld-Jorgensen EC. Effects of perfluoroalkyl acids on the function of the thyroid hormone and the aryl hydrocarbon receptor. Environ Sci Pollut Res. 2013;20: 8045-8056. doi: 10.1007/s11356-013-1628-7.
18. Jin R, McConnell R, Catherine C, et al. Perfluoroalkyl substances and severity of nonalcoholic fatty liver in Children: An untargeted metabolomics approach. Environ Int. 2020;134:105220. doi: 10.1016/j.envint.2019.105220.
19. Marchese MJ, Zhu T, Hawkey AB, et al. Prenatal and perinatal exposure to Per- and polyfluoroalkyl substances (PFAS)-contaminated drinking water impacts offspring neurobehavior and development. Sci Total Environ. 2024;917:170459. doi: 10.1016/j.scitotenv.2024.170459.
20. Wright JM, Lee AL, Rappazzo KM, Ru H, Radke EG, Bateson TF. Systematic review and meta-analysis of birth weight and PFNA exposures. Environ Res. 2023;222:115357. doi: 10.1016/j.envres.2023.115357.
21. Gorrochategui E, Perez-Albaladejo E, Casas J, Lacorte S, Porte C. Perfluorinated chemicals: Differential toxicity, inhibition of aromatase activity and alteration of cellular lipids in human placental cells. Toxicol Appl Pharmacol. 2014;277:124-130. doi: 10.1016/j.taap.2014.03.012.
22. Ren X-M, Qin W-P, Cao L-Y, et al. Binding interactions of perfluoroalkyl substances with thyroid hormone transport proteins and potential toxicological implications. Toxicol. 2016;366-367:32-42. doi: 10.1016/j.tox.2016.08.011.
23. Wang T, Lin Z, Yin D, Tian D, Zhang Y, Kong D. Hydrophobicity-dependent QSARs to predict the toxicity of perfluorinated carboxylic acids and their mixtures. Environ Toxicol Pharmacol. 2011;32(2):259-265. doi: 10.1016/j.etap.2011.05.011.
24. Zhang L, Ren X-M, Guo L-H. Structure-based investigation on the interaction of perfluorinated compounds with human liver fatty acid binding protein. Environ Sci Technol. 2013;47:11293-11301. doi: 10.1021/es4026722.
25. Amstutz VH, Sijm DTHM, Vrolijk MF. Perfluoroalkyl substances and immunotoxicity: An in vitro structure-activity relationship study in THP-1-derived monocytes and macrophages. Chemosphere 2024;364:143075. doi: 10.1016/j.chemosphere.2024.143075.
26. Vanden Heuvel JP. Perfluorodecanoic acid as a useful pharmacologic tool for the study of peroxisome proliferation. Gen Pharmacol. 1996;27(7):1123-1129. doi: 10.1016/0306-3623(95)00126-3.
27. Luz AL, Anderson JK, Goodrum P, Durda J. Perfluorohexanoic acid toxicity, part I: Development of a chronic human health toxicity value for use in risk assessment. Regul Toxicol Pharmacol. 2019;103:41-55. doi: 10.1016/j.yrtph.2019.01.019.
28. Anderson JK, Luz AL, Goodrum P, Durda J. Perfluorohexanoic acid toxicity, part II: Application of human health toxicity value for risk characterization. Regul Toxicol Pharmacol. 2019;103:10-20. doi: 10.1016/j.yrtph.2019.01.020.
29. Holder C, DeLuca N, Luh J, et al. Systematic evidence mapping of potential exposure pathways for per- and polyfluoroalkyl substances based on measured occurrence in multiple media. Environ Sci Technol. 2023;57(13):5107-5116. doi: 10.1021/acs.est.2c07185.
30. Vakili M, Bao Y, Gholami F, et al. Removal of HFPO-DA (GenX) from aqueous solutions: a mini-review. Chem Eng J. 2021;424:130266. doi: 10.1016/j.cej.2021.130266.
31. Baggioli A, Sansotera M, Navarrini W. Thermodynamics of aqueous perfluorooctanoic acid (PFOA) and 4,8-dioxa-3H-perfluorononanoic acid (DONA) from DFT calculations: Insights into degradation initiation. Chemosphere. 2018;193:1063-1070. doi: 10.1016/j.chemosphere.2017.11.115.
32. Shearer JJ, Callahan CL, Calafat AM, et al. Serum concentrations of per- and polyfluoroalkyl substances and risk of renal cell carcinoma. J Natl Cancer Inst. 2021;113(5):580-587. doi: 10.1093/jnci/djaa143.
33. Purdue MP, Rhee J, Denic-Roberts H, et al. A nested case–control study of serum per- and polyfluoroalkyl substances and testicular germ cell tumors among U.S. Air Force servicemen. Environ Health Perspect. 2023;131(7):077007. doi: 10.1289/EHP12603.
34. Rhee J, Barry KH, Huang W-Y, et al. A prospective nested case-control study of serum concentrations of per- and polyfluoroalkyl substances and aggressive prostate cancer risk. Environ Res. 2023;228:115718. doi: 10.1016/j.envres.2023.115718.
35. Chang VC, Rhee J, Berndt SI, et al. Serum perfluorooctane sulfonate and perfluorooctanoate and risk of postmenopausal breast cancer according to hormone receptor status: An analysis in the Prostate, Lung, Colorectal and Ovarian Cancer Screening Trial. Int J Cancer. 2023;153(4):775-782. doi: 10.1002/ijc.34487.
36. Yang W, Ling X, He S, et al. PPARα/ACOX1 as a novel target for hepatic lipid metabolism disorders induced by per- and polyfluoroalkyl substances: An integrated approach. Environ Int. 2023;178:108138. doi: 10.1016/j.envint.2023.108138.
37. Costello E, Rock S, Stratakis N, et al. Exposure to per- and polyfluoroalkyl substances and markers of liver injury: A systematic review and meta-analysis. Environ Health Perspect. 2022;130(4):046001. doi: 10.1289/EHP10092.
38. Goodrich JA, Walker D, Lin X, et al. Exposure to perfluoroalkyl substances and risk of hepatocellular carcinoma in a multiethnic cohort. JHEP Rep. 2022;4(10):100550. doi: 10.1016/j.jhepr.2022.100550.
39. van Gerwen M, Colicino E, Guan H, et al. Per- and polyfluoroalkyl substances (PFAS) exposure and thyroid cancer risk. EBioMedicine. 2023;97:104831. doi: 10.1016/j.ebiom.2023.104831.
40. Sohn YS, Park YJ, Oh CM. Effect of multiple exposure to perfluorinated chemicals on thyroid function among adults in the US: The National Health and Nutrition Examination Survey 2007-2008 and 2011-2012. Int J Thyroidol. 2020;13(1):19-29. doi: 10.11106/ijt.2020.13.1.19.
41. Taylor KD, Woodlief TL, Ahmed A, Hu Q, Duncker PC, DeWitt JC. Quantifying the impact of PFOA exposure on B-cell development and antibody production. Toxicol Sci. 2023;194(1):101-108. doi: 10.1093/toxsci/kfad043.
42. Wang L-Q, Liu T, Yang S, et al. Perfluoroalkyl substance pollutants activate the innate immune system through the AIM2 inflammasome. Nat Commun. 2021;12(1):2915. doi: 10.1038/s41467-021-23201-0.
43. Britton KN, Judson RS, Hill BN, et al. Using zebrafish to screen developmental toxicity of per- and polyfluoroalkyl substances (PFAS). Toxics. 2024;12(7):501. doi: 10.3390/toxics12070501.
44. Raymer JH, Michael LC, Studabaker WB, et al. Concentrations of perfluorooctane sulfonate (PFOS) and perfluorooctanoate (PFOA) and their associations with human semen quality measurements. Reprod Toxicol. 2012;33(4):419-427. doi: 10.1016/j.reprotox.2011.05.024.
45. Oh J, Schmidt RJ, Tancredi D, et al. Prenatal exposure to per- and polyfluoroalkyl substances and cognitive development in infancy and toddlerhood. Environ Res. 2021;196:110939. doi: 10.1016/j.envres.2021.110939.
46. Environmental Protection Agency. Laws and regulations. EPA. Accessed February 16, 2025. https://www.epa.gov/laws-regulations.
47. European Chemicals Agency. Per- and polyfluoroalkyl chemicals (PFAS). ECHA. Accessed February 16, 2025. https://echa.europa.eu/hot-topics/perfluoroalkyl-chemicals-pfas
48. Environmental Protection Agency. Method 537.1: Determination of selected per- and polyfluorinated alkyl substances in drinking water by solid phase extraction and liquid chromatography/tandem mass spectrometry (LC/MS/MS). EPA. https://cfpub.epa.gov/si/si_public_record_report.cfm?dirEntryId=343042&Lab=NERL. Published November 2018. Accessed February 16, 2025.
49. Environmental Protection Agency. Method 533: Determination of per- and polyfluoroalkyl substances in drinking water by isotope dilution anion exchange solid phase extraction and liquid chromatography/tandem mass spectrometry. EPA. https://www.epa.gov/dwanalyticalmethods/method-533-determination-and-polyfluoroalkyl-substances-drinking-water-isotope. Published December 2019. Accessed February 16, 2025.
50. Environmental Protection Agency. SW-846 test method 8327: Per- and polyfluoroalkyl substances (PFAS) by liquid chromatography/tandem mass spectrometry (LC/MS/MS). EPA. https://www.epa.gov/hw-sw846/sw-846-test-method-8327-and-polyfluoroalkyl-substances-pfas-liquid-chromatographytandem. Published June 2021. Accessed February 16, 2025.
51. Environmental Protection Agency. Draft method 1633: Analysis of per- and polyfluoroalkyl substances (PFAS) in aqueous, solid, biosolids, and tissue samples by LC-MS/MS. EPA. https://www.epa.gov/system/files/documents/2021-09/method_1633_draft_aug-2021.pdf. Published August 2021. Accessed February 16, 2025.
52. Environmental Protection Agency. Other test method 45 (OTM-45): Measurement of selected per- and polyfluoroalkyl substances (PFAS) from stationary sources. EPA. https://www.epa.gov/sites/default/files/2021-01/documents/otm_45_semivolatile_pfas_1-13-21.pdf. Published January 13, 2021. Accessed February 16, 2025.
53. U.S. Food and Drug Administration. Testing food for PFAS and assessing dietary exposure. FDA. https://www.fda.gov/food/process-contaminants-food/testing-food-pfas-and-assessing-dietary-exposure. Published July 20, 2023. Accessed February 16, 2025.
54. Menger RF, Beck JJ, Borch T, Henry CS. Colorimetric paper-based analytical device for perfluorooctanesulfonate detection. ACS ES&T Water. 2022;2(4):565-572. doi: 10.1021/acsestwater.1c00356.
55. Clark RB, Dick JE. Towards deployable electrochemical sensors for per- and polyfluoroalkyl substances (PFAS). Chem Commun. 2021;57(66):8121-8130. doi: 10.1039/D1CC02641K.
Concellón A, Castro-Esteban J, Swager TM. Ultratrace PFAS detection using amplifying fluorescent polymers. J Am Chem Soc. 2023;145(20):11420-11430. doi: 10.1021/jacs.3c03125