The Role of Stable Isotope Tracers in Skeletal Muscle Metabolism Research
Stable isotope tracers help scientists track metabolism, study muscle protein synthesis and uncover key insights into human health.
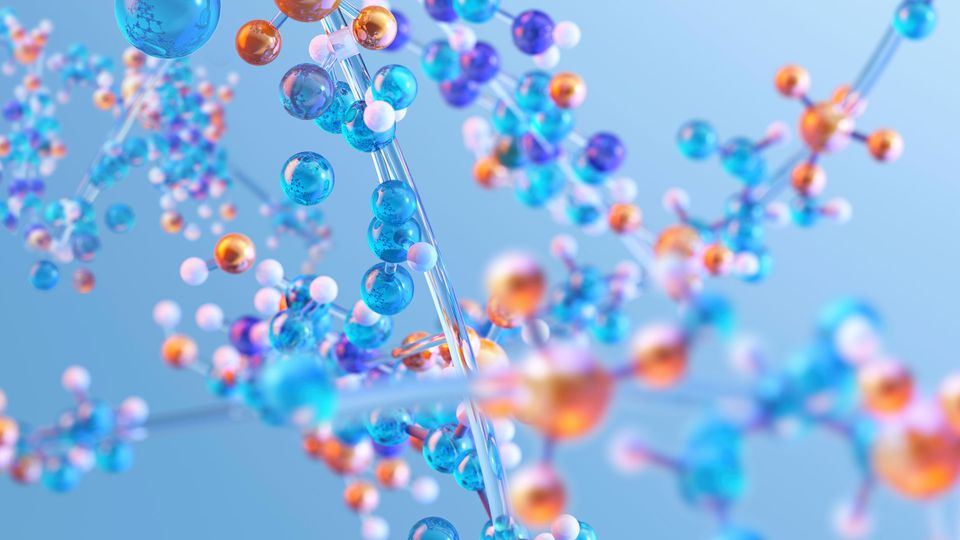
Complete the form below to unlock access to ALL audio articles.
Isotopes are forms of a chemical element that contain the same number of protons and electrons but have a different number of neutrons. In general, the lighter isotope of an element tends to be more abundant in nature – for example, 98.9% of the Earth’s carbon is 12C, with 13C making up the remaining 1.1%.
Although they are chemically and functionally identical, a difference in mass makes isotopes analytically detectable – typically performed by mass spectrometry (MS) which separates atoms or molecules based on their mass and/or charge.1 When the heavier isotope is introduced into a biological system, it is treated in the same way as the endogenous lighter compound. However, the presence of the heavy isotope “label” allows us to “trace” the metabolism of this compound within the system.2
Generally, stable isotope tracers are substrate-specific. Labeled fatty acids are used to assess fat metabolism, deuterated glucose tracers are used to measure carbohydrate metabolism and labeled amino acid (AA) tracers are used to assess protein metabolism.
The use of AA tracers has allowed us to uncover complex interactions between muscle protein synthesis (MPS) and muscle protein breakdown (MPB) that are needed to maintain skeletal muscle mass and what happens when this dynamic equilibrium is disturbed.1
Intravenous infusion of AA stable isotope tracers
“Skeletal muscle proteins are made up of long chains of individual AAs and are continually being broken down and synthesized,” Dr. Emily Lantz, assistant professor in the Department of Nutrition Sciences and Health Behavior at the University of Texas Medical Branch, told Technology Networks. “To understand how the rate of muscle protein synthesis changes in response to differing stimuli, we can use the traditional intravenous (IV)-infused stable isotope tracer method.”
Traditional stable isotope tracer experiments require sterile infusates, IV cannulation and multiple blood and tissue samples. These constant IV infusions require subjects to be studied in a controlled clinical or laboratory environment, restricting measures to <24 hours and traditionally over 2–12 hours – an inherent limitation of this technique.
Although the time-limited, invasive nature of these studies narrows their application and may not translate to chronic settings, these substrate-specific tracer techniques have been invaluable to our understanding of human metabolism.
Using this technique, muscle protein metabolism can be determined in two main ways: arteriovenous (AV) balance and direct incorporation methods (i.e., the precursor product technique).
The AV balance technique looks at the change in the concentration of the tracer across a whole limb (usually the leg) to estimate muscle protein synthesis, breakdown and net balance. Lantz detailed, “To do this, an IV infusion of a stable isotope tracer (e.g., labeled AA) is combined with arterial blood sampling to measure the concentration of the labeled AA, and the appearance of the unlabeled AA (tracee) from the muscle in the vein, which provides an approximation of MPB.”
Compared to AV balance, the precursor-product technique is generally considered the “gold standard” for measuring skeletal muscle protein turnover/or fractional synthetic rate due to the detailed mechanistic understanding it provides.
When the labeled AA (i.e., the precursor) enters the intracellular AA pool of a muscle cell, it will eventually be incorporated into newly synthesized proteins within that tissue (i.e., the product). By sampling these tissues and measuring the amount of incorporated labeled AA over time with reference to the amount of tracer in the precursor pool (intracellular labeled AA, aminoacyl-tRNA or a surrogate such as α-ketoisocaproate enrichment), a rate of synthesis can be determined.3
The AV balance method can be expanded with the precursor product technique into what is called the three-pool model. This includes measurement of tracer/tracee enrichments and concentrations in the femoral artery and vein, as well as a muscle biopsy to look at free tracee enrichment in the intracellular pool. Using this method allows for the direct calculation of MPS and MPB of a specific muscle rather than an estimate across the whole limb.
“Both techniques are technically challenging due to the muscle biopsies, isotope infusion and repeated blood samples. The AV balance method is particularly challenging because it requires catheterization of the femoral artery and vein as well as measurement of blood flow,” Lantz notes.
The resurgence of deuterium oxide
Over the last few years, using deuterium oxide (D2O), or “heavy water”, to measure MPS over longer periods in free-living environments has gained traction. Compared to traditional IV-infused tracers, D2O is much less invasive, with no catheterization and less frequent blood draws and muscle biopsies.
“Participants drink a bolus of D2O which has a labeled hydrogen (2H). The 2H quickly equilibrates with the body water pool and is eventually incorporated into endogenously synthesized alanine (via transamination of pyruvate),” said Lantz. “The 2H-labeled alanine is available to be incorporated into tissue proteins (including skeletal muscle), which can be assessed over several days or weeks to measure changes in protein synthesis in response to nutrition, physical activity or disease,” she added.
D2O has a long half-life within the body water pool of ∼9–11 days; therefore, the use of a single bolus with daily or weekly top-up loading strategies permits these longer measurements.
Although usually used for chronic assessment, D2O can also be used to assess acute MPS. This was shown by Brook and colleagues, who compared the use of D2O over 3–6 hours to the classically used substrate-specific tracer, ring-[13C6]-phenylalanine. Measurement of MPS over 3 hours in both postabsorptive and postprandial (20 g oral of essential AA) states revealed comparable mean fractional synthetic rates and magnitudes of change in feeding responses.4 As such, the use of D2O has shown great efficacy in measurements of MPS over days, weeks and months and in the investigation of muscle metabolism.
Intrinsically labeled protein provides an extra level of detail
An even more comprehensive assessment of postprandial (i.e., post-feeding) protein handling can be achieved by combining the infusion of stable isotope-labeled AAs with the ingestion of intrinsically labeled protein, alongside frequent blood and muscle samples.5
Intrinsically labeled proteins can be produced “by feeding chickens a stable isotope to have a labeled egg, cows can produce labeled milk or meat, or plants can be watered with D2O to produce a labeled plant protein,” explained Lantz.
Intrinsically labeled milk protein was first obtained by Boirie et al. by infusing a lactating cow with L-[1-13C]-leucine, which resulted in the incorporation of L-[1-13C]-leucine into milk protein.6 The intrinsically labeled milk was collected and subsequently ingested by human participants. Blood sample collection and analysis allowed the in vivo assessment of the post-prandial appearance rate of milk protein-derived L-[1-13C]-leucine into the circulation.7, 8
“The advantage of this technique is that it more closely models how AAs that we consume in our diet are absorbed by our body following a meal and the bioavailability to be incorporated into skeletal muscle,” said Lantz. “However, while this is another powerful technique, it is also technically challenging and very expensive to produce the intrinsically labeled protein.”
MS allows for the quantification of muscle protein metabolism
Often, the choice of AA tracer and study design are directed by the analytical sensitivity of the MS equipment at hand. Typically, AAs are separated from other compounds and molecules within the collected blood plasma and/or muscle tissue via some form of chromatography – traditionally gas chromatography (GC) but now, more commonly, liquid chromatography (LC) – before being introduced into a mass spectrometer for identification and analyses.
With standard single quadrupole GC/LC-MS, accurate detection of isotopic abundance is limited to ∼0.1 atom percent excess – equivalent to having 0.1% more heavy isotope than normal in the system. However, detection levels can be improved fivefold to ∼0.0005% when GC/LC is combined with combustion–isotope ratio MS techniques.9
This is particularly important when measuring the metabolic pools that turn over at a slow rate, such as that of skeletal muscle protein which has a turnover rate of ∼1–1.5%/day in an adult human, and in systems or populations where it might not be possible or economical to add large amounts of a stable isotope tracer to reach such high levels of enrichment, such as the study of human metabolism.10
“One of the more recent advances in MS allows for better resolution in the application of D2O methodology to determine the rate of turnover of pools of protein that turn over quickly or more slowly,” said Lantz.
AA tracers helped reveal how and why muscle declines over time
Sarcopenia is the age-related loss of muscle mass, strength and function. This progressive decline in muscle tissue is particularly pronounced in older adults, typically those over the age of 70, who exhibit 10–30% less muscle mass compared to their younger, healthier counterparts. Such reductions in muscle mass have profound consequences for physical function, metabolic health and overall quality of life.
The underlying cause of sarcopenia is a gradual, age-associated decline in muscle mass, estimated at approximately 1% per year after the age of 50.11 This decline results from a chronic imbalance between MPS and MPB. Notably, postabsorptive (fasting) MPS and MPB rates remain largely unchanged with aging. Consequently, the loss of muscle over time is not due to an increase in MPB but rather a diminished capacity to respond to anabolic stimuli that typically maintain muscle mass in youth.
Seminal research by Cuthbertson et al. (2005) provided crucial insights into this phenomenon. 12 By directly comparing MPS responses to essential AA intake in young and older individuals, the researchers demonstrated that the ability to stimulate MPS in response to protein intake is blunted with aging. This impaired anabolic response, termed "anabolic resistance," leads to a reduced capacity to replenish muscle lost during fasting periods. Over time, this disruption in muscle homeostasis results in net muscle protein loss, thereby accelerating sarcopenia.
Moreover, anabolic resistance extends beyond protein feeding and affects other key anabolic stimuli. For example, older adults exhibit impaired muscle anabolic responses to insulin and resistance exercise, which are critical for maintaining muscle mass. 13, 14 These deficits further contribute to the progressive nature of sarcopenia and highlight the complexity of its underlying mechanisms.
Contemporary research continues to explore strategies to mitigate sarcopenia by leveraging stable isotope tracer methodologies to assess muscle protein metabolism “to personalize protein needs in healthy and diseased individuals to optimize muscle anabolism,” Lantz concluded.
- Wilkinson DJ, Brook MS, Smith K, Atherton PJ. Stable isotope tracers and exercise physiology: past, present and future. J Physiol. 2017;595(9):2873-2882. doi: 10.1113/JP272277
- Brook MS, Wilkinson DJ. Contemporary stable isotope tracer approaches: Insights into skeletal muscle metabolism in health and disease. Exp Physiol. 2020;105(7):1081-1089. doi: 10.1113/EP087492
- Watt PW, Lindsay Y, Scrimgeour CM, et al. Isolation of aminoacyl-tRNA and its labeling with stable-isotope tracers: Use in studies of human tissue protein synthesis. PNAS. 1991;88(13):5892-5896. doi: 10.1073/pnas.88.13.5892
- Wilkinson DJ, Cegielski J, Phillips BE, et al. Internal comparison between deuterium oxide (D2O) and L-[ring-13C6] phenylalanine for acute measurement of muscle protein synthesis in humans. Physiol Rep. 2015;3(7):e12433. doi: 10.14814/phy2.12433
- Trommelen J, Holwerda AM, Pinckaers PJM, Loon LJC van. Comprehensive assessment of post-prandial protein handling by the application of intrinsically labelled protein in vivo in human subjects. Proc Nutr Soc. 2021;80(2):221-229. doi: 10.1017/S0029665120008034
- Boirie Y, Fauquant J, Rulquin H, Maubois JL, Beaufrère B. Production of large amounts of [13C]leucine-enriched milk proteins by lactating cows. J Nutr. 1995;125(1):92-98. doi: 10.1093/jn/125.1.92
- Boirie Y, Dangin M, Gachon P, Vasson MP, Maubois JL, Beaufrère B. Slow and fast dietary proteins differently modulate postprandial protein accretion. PNAS. 1997;94(26):14930-14935. doi: 10.1073/pnas.94.26.14930
- van Loon LJC, Boirie Y, Gijsen AP, et al. The production of intrinsically labeled milk protein provides a functional tool for human nutrition research1. JDS. 2009;92(10):4812-4822. doi: 10.3168/jds.2009-2317
- Wilkinson DJ. Historical and contemporary stable isotope tracer approaches to studying mammalian protein metabolism. Mass Spectrom Rev. 2018;37(1):57-80. doi: 10.1002/mas.21507
- Wilkinson DJ, Franchi MV, Brook MS, et al. A validation of the application of D2O stable isotope tracer techniques for monitoring day-to-day changes in muscle protein subfraction synthesis in humans. Am J Physiol Endocrinol. 2014;306(5):E571-E579. doi: 10.1152/ajpendo.00650.2013
- Mitchell WK, Atherton PJ, Williams J, Larvin M, Lund JN, Narici M. Sarcopenia, dynapenia, and the impact of advancing age on human skeletal muscle size and strength; a quantitative review. Front Physiol. 2012;3. doi: 10.3389/fphys.2012.00260
- Cuthbertson D, Smith K, Babraj J, et al. Anabolic signaling deficits underlie amino acid resistance of wasting, aging muscle. The FASEB Journal. 2005;19(3):1-22. doi: 10.1096/fj.04-2640fje
- Wilkes EA, Selby AL, Atherton PJ, et al. Blunting of insulin inhibition of proteolysis in legs of older subjects may contribute to age-related sarcopenia12. AJCN. 2009;90(5):1343-1350. doi: 10.3945/ajcn.2009.27543
- Kumar V, Selby A, Rankin D, et al. Age-related differences in the dose–response relationship of muscle protein synthesis to resistance exercise in young and old men. J Physiol. 2009;587(1):211-217. doi: 10.1113/jphysiol.2008.164483