Transform MSC Research With Real-Time, Non-Invasive Differentiation Monitoring
App Note / Case Study
Published: February 12, 2025
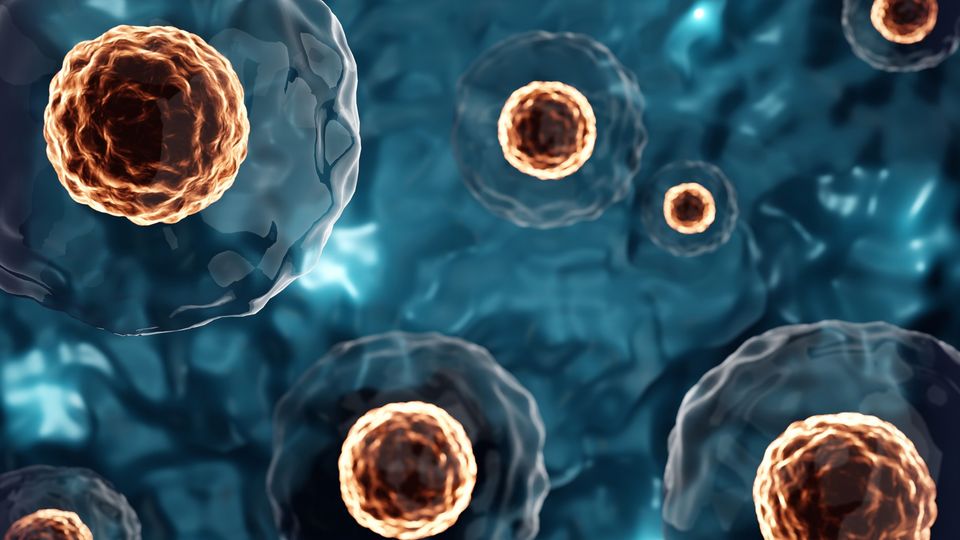
Credit: iStock
Researchers studying mesenchymal stem cells (MSCs) face significant challenges with traditional endpoint assays that provide only limited snapshots of the differentiation process.
The inability to monitor differentiation in real time can result in missed insights and inefficient use of valuable cellular material.
This application note demonstrates how combining live-cell analysis with high-throughput screening cytometry enables continuous, non-invasive monitoring of MSC differentiation, offering unprecedented insights into cellular dynamics while preserving samples for further analysis.
Download this application note to learn:
- How to implement a comprehensive workflow for real-time MSC differentiation monitoring
- Advantages of combining live-cell imaging with high-throughput cytometry analysis
- Strategies for achieving more reliable and reproducible MSC functionality assessments
Application Note
Rapid Assessment of MSC Differentiation with
Non-Invasive Monitoring and HTS Cytometry
Authors: Natasha Lewis, Jasmine Trigg, Daryl Cole, Nicola Bevan
Sartorius UK Ltd, Royston, Hertfordshire, UK
Correspondence
Email: askascientist@sartorius.com
Abstract
Mesenchymal stem cells (MSCs) are adult stem cells with multipotent differentiation potential and significant
immunomodulatory capacity, making them highly valuable in regenerative medicine, tissue engineering, and cell therapy.
Ensuring the therapeutic efficacy of MSCs necessitates rigorous confirmation of their differentiation potential into specific cell
types, such as osteoblasts and adipocytes. Traditional endpoint assays for assessing MSC differentiation are limited in providing
dynamic insights, highlighting the need for advanced technologies that offer real-time, high-throughput, and multiparametric
analysis. This study utilizes the Incucyte® Live-Cell Analysis System and iQue® High-Throughput Screening Cytometer to
monitor MSC differentiation in real-time. These technologies enable continuous live-cell imaging and rapid, multiplexed analysis,
offering a comprehensive view of the differentiation process. The results demonstrate quicker confirmation of differentiation
potential and preservation of cellular material for further analysis, enhancing the efficiency and reliability of MSC research.
September 2024
Keywords or phrases:
Mesenchymal stem cells, Differentiation,
Cell therapy, Tissue engineering, Live-cell analysis,
Osteogenesis, Adipogenesis
For further information, visit sartorius.com
2
Introduction
Mesenchymal stem cells (MSCs) are adult stem cells resident
predominantly in the bone marrow alongside other sites
including adipose tissue, umbilical cord blood, and dental
pulp. They are self-renewing and have differentiation
potential to the chondrocyte, osteocyte, and adipocyte
lineages. MSCs have known capacities in immunomodulation
and paracrine effects that stimulate tissue repair and
regeneration. Their multi-potency and functionality make
them of great interest in the fields of regenerative medicine,
tissue engineering, and cell therapy, both in terms of direct
repair and for treating inflammatory and autoimmune
diseases. As the body of research into these unique cells
grows, so does their medical potential. Clinical examples of
the use of MSCs include treatment of osteoarthritis,¹ graft
versus host disease,² and acute myocardial infarction.³
The therapeutic efficacy of MSCs is critically dependent on
their differentiation potential into specific cell types, which is
a highly regulated process influenced by various biochemical
and physical cues. Osteogenic differentiation leads to the
formation of osteoblasts, which are essential for bone
formation and remodeling. Differentiation promoting factors
elicit the expression of osteogenic markers including alkaline
phosphatase (ALPL) and extracellular deposition of calcium
phosphate (hydroxyapatite). Adipogenesis leads to the
formation of adipocytes which play a vital role in tissue
homeostasis by storing energy as fat. As such, key features of
adipocytes include characteristic cytoplasmic lipid droplets,
a rounded morphology, and expression of adipogenic
markers and adipokines.
The ability of MSCs to differentiate must be rigorously
confirmed before they can be utilized in downstream
applications, as it is a key characteristic used for their
identification.⁴ Traditional methods for assessing MSC
differentiation are often endpoint assays, which provide
limited insights into the dynamic processes of differentiation.
This limitation underscores the need for advanced
technologies that can offer real-time, high-throughput,
and multiparametric analysis.
Addressing these challenges requires the development
and adoption of standardized, reliable, and reproducible
methods for MSC differentiation assessment, using
advanced technologies such as the Incucyte® Live-Cell
Analysis System (Incucyte® System) and iQue® HighThroughput Screening (HTS) Cytometer. Continuous livecell imaging allows researchers to monitor morphological
changes and differentiation markers over time. This method
provides valuable insights into the kinetics of cell
differentiation, proliferation, and morphology, offering a
dynamic view of cellular processes that static endpoint
assays cannot capture. Meanwhile, HTS by cytometry
facilitates rapid, multiplexed analysis of cellular phenotypes
and secreted factors, providing a comprehensive view of
the differentiation process. The iQue® platform’s highthroughput capabilities allow for the simultaneous analysis of
multiple parameters across large sample sets, significantly
enhancing the efficiency and depth of MSC research.
Here, we present a comprehensive workflow to evaluate
the functionality of MSCs. This workflow aims to provide
robust and reliable methods for assessing MSC
differentiation, thereby enhancing the reliability and
efficiency of experimental workflows and ensuring the
therapeutic potential of MSCs is fully realized.
3
Materials and Methods
Media for Routine Cell Culture and for Differentiation
Materials Supplier Cat. No. Final Concentration
MSC NutriStem® XF Medium Sartorius 05-200-1A
MSC NutriStem® XF Supplement Mix Sartorius 05-201-1U
MSCgo™ Osteogenic Differentiation Medium Sartorius 05-440-1B
MSCgo™ Rapid Osteogenic Differentiation Medium Sartorius 05-442-1B
MSCgo™ Adipogenic SF, XF Supplement Mix I Sartorius 05-331-1-01
MSCgo™ Adipogenic SF, XF Supplement Mix II Sartorius 05-332-1-15
NutriCoat™ Attachment Solution Sartorius 05-760-1-15 1:500
Incucyte® Opti-Green Reagent (supplied with Incucyte® Mouse IgG1
Fabfluor Antibody Labeling Dye)
Sartorius 4745 0.5 µM
Calcein Merck CO875 1 µM
BioTracker 488 Green Lipid Droplet Dye Merck SCT144 1:1,000
Bone marrow mesenchymal stem cells RoosterVial™-hBM RoosterBio MSC-003
Table 1: Cell culture media and reagents used for culturing MSCs.
Surface Marker Expression Panel Reagents
Marker Product Name Supplier Cat. No. Final Concentration
Viability Zombie Violet™
Fixable Viability Kit
BioLegend 423113 1 :200
MSC marker panel CD34 FITC anti-human CD34
Antibody
Invitrogen MA5-16925 1:100
CD45 Brilliant Violet 570™ antihuman CD45 Antibody
BioLegend 304033 1:100
CD73 APC anti-human CD73
(Ecto-5'-nucleotidase)
Antibody
BioLegend 344005 1:100
CD90 PE/Dazzle™ 594 anti-human
CD90 (Thy1) Antibody
BioLegend 328133 1:100
CD105 PE/Cyanine7 anti-human
CD105 Antibody
BioLegend 323217 1:100
Fixative Fixation buffer BioLegend 420801 1X
Permeabilization
buffer
Intracellular Staining Perm
Wash Buffer (10X)
BioLegend 421002 1X
Osteogenesis
marker
Alkaline phosphatase
(ALPL)
Human Alkaline
Phosphatase/ALPL
PE-conjugated Antibody
R&D Systems FAB1448P-025 1:100
Adipogenesis
marker
Fatty Acid Binding
Protein 4 (FABP4)
Alexa Fluor® 647
Anti-FABP4 antibody
Abcam ab216529 1:100
Table 2: Reagents used for cell analysis using the iQue® Cytometer.
4
MSC Culture
Prior to seeding into culture vessels for routine culture and
experiments, plates and flasks were pre-coated with
NutriCoat™ Attachment Solution. The solution was diluted in
PBS according to the recommended volumes, added to the
culture vessel and incubated for 1 hour in a humidified CO₂
incubator (37°C). Prior to cell seeding, the solution was
aspirated, and cell culture medium containing a cell
suspension was quickly added to ensure that the coating did
not dry out.
MSCs were obtained from RoosterBio and cultured in MSC
Nutristem® XF basal medium (basal medium). They were
seeded at a density of 3,000 cells/cm² in tissue culture flasks,
passaged at 80% confluency and analyzed using the
Incucyte® System.
MSC Differentiation
MSCs were differentiated into osteoblasts using either
MSCgo™ Osteogenic Differentiation Medium or MSCgo™
Rapid Osteogenic Differentiation Medium. Briefly, MSCs
were seeded into 24-well plates pre-coated with MSC
attachment solution at 6 × 10⁴ cells/well (3 × 10⁴/cm²) in basal
medium. After 24 hours, or when the cells had reached 80%
confluency, the medium was exchanged to differentiation
medium containing Incucyte® Opti-Green (background
suppressor) and calcein. Cells were cultured in differentiation
medium for either 21 or 10 days, respectively.
MSCs were differentiated into adipocytes using MSCgo™
Adipogenic Differentiation Medium. Briefly, MSCs were
seeded into 24-well plate pre-coated with MSC attachment
solution at 6 × 10⁴ cells/well (3 × 10⁴/cm²) in basal medium.
After 24 hours or when the cells had reached 80%
confluency, the medium was exchanged to differentiation
medium containing Incucyte® Opti-Green and BioTracker
488 Green Lipid Droplet Dye. Following 12 days of culture,
the medium was exchanged to complete basal medium, and
the cells were cultured for a further 4 days.
Throughout differentiation, high-definition phase-contrast
and green fluorescence images were acquired using the
Incucyte® System and analyzed using integrated software.
Label-free quantification was performed using the Incucyte®
AI Cell Health Analysis Software Module, which enables
AI-based segmentation of heterogenous cell morphologies
to give univariate morphology metrics (AI Cell
Segmentation),
Surface Marker Expression
At regular time points throughout differentiation, cells were
stained for viability and with the MSC marker panel (CD34,
CD45, CD73, CD90, and CD105) as well as specific lineage
differentiation markers. Briefly, following harvest using
trypsin, cells were washed in PBS and stained for viability
using Zombie Violet™ for 20 minutes. The cells were seeded
into a 96-well V-bottom plate at 20K/well and washed twice
with PBS + 2% FBS before staining with the MSC marker
antibody cocktail as described in Table 2 for 30 minutes at
room temperature. The cells were washed twice by
centrifugation (5 min at 1,000 × g) using PBS + 2% FBS. Cells
were then resuspended in 20 µl of PBS + 2% FBS and
analyzed on the iQue® HTS cytometer.
For ALPL, a surface marker, live cells were stained with the
ALPL antibody in parallel to the marker panel. For
intracellular Fatty Acid Binding Protein 4 (FABP4) staining,
cells were fixed using fixation buffer for 20 minutes at room
temperature, washed twice in permeabilization buffer, and
then stained with FABP4 antibody in PBS + 2% FBS for
30 minutes at room temperature. Cells were washed twice by
centrifugation before being analyzed using the iQue®
Platform. Data was analyzed using integrated iQue Forecyt®
Software.
Results
Real-Time Quantification of Osteogenic Differentiation
A key indicator of MSC quality is the ability to differentiate into
cells of multiple lineages, including osteoblasts. Osteogenic
differentiation is typically evaluated using an endpoint
histological stain for mineralized calcium deposits such as Von
Kossa or Alizarin Red S staining. However, these differentiation
experiments can typically last for 21 days before the
differentiation can be confirmed, the staining process can be
time consuming and labour-intensive, they require
destruction of the cell samples, and they are typically only
semi-quantitative without further laborious analysis steps.
A live-cell solution would allow quicker confirmation of the
differentiation potential, insight into the dynamics of the
differentiation process, and preservation of precious cellular
material for further evaluation including surface marker
analysis.
Here, we demonstrate a simple assay for live-cell
quantification of osteogenic differentiation in real-time.
Calcein (not to be confused with the viability stain calcein AM)
binds to the calcium in growing calcium phosphate and
calcium carbonate crystals, which are extracellularly deposited
as cells are directed to the bone lineage. As calcein can be
incubated with cells for the duration of an experiment, it can
be used for continuous assessment of mineralization, and in
3D cultures has been used as a superior alternative to Alizarin
Red.⁵
B
Calcein (Total area) x10⁵
Time (days)
0
5
10
15
20
0 2 4 6 8 10 12 14 16 18 20
Basal medium
Osteogenic
Rapid osteogenic
Basal medium
Osteogenic
Rapid Osteogenic
0d
0d
0d
0d
0d
0d
2d
2d
2d
2d
3d
3d
10d
10d
10d
10d
9d
9d
A
5
Figure 1: Fluorescent Evaluation of Osteogenic Differentiation of MSCs Cultured in Osteogenic Differentiation Medium
Using Live-Cell Analysis. (A) Representative fluorescence and phase-contrast images of MSCs cultured in osteogenic
medium, rapid osteogenic medium or MSC basal medium with calcein at intervals during a 21-day period. (B) Quantification
of fluorescent calcein signal from images captured during the differentiation period.
A
CD90 CD73 CD105 CD45 CD34
0
20
40
60
80
100
120
Marker
Percentage positive (%)
Day 2
Day 4
Day 6
Day 9
Day 11
Day 17
B
2 4 6 9 11 13 16 17 21
0
20
40
60
80
100
ALPL
Time (days)
Percentage positive (%)
Basal medium
Osteogenic
Rapid osteogenic
6
Figure 1A demonstrates that osteogenic differentiation can
be fluorescently detected as early as 2 days, as indicated by
the appearance of green fluorescent deposits within the
culture at that time point. In contrast, no fluorescent signal
was observed in the control condition. There was also a visual
difference in the morphology of cells cultured in osteogenic
medium compared to those in basal medium, although it was
more subtle and the mineral deposits were only distinct at
the later time points. Thus, the calcein stain allows a robust,
non-perturbing and clear early detection method for
evaluating osteogenic differentiation.
Rapid osteogenic medium offers faster differentiation of
osteoblasts, with a differentiation timescale of 15 days rather
than 21 days. Similar to the osteogenic medium, calcium
fluorescence was observable in cells cultured in the rapid
differentiation medium 3 days post-culture initiation
(Figure 1A). The mineral deposition was visible by eye after
6 days, and the pattern of deposition was slightly more
regular than in the osteogenic medium.
Figure 1B depicts the quantification of this fluorescent signal
throughout the differentiation period. In cells cultured in
osteogenic media, there was an evident increase in the total
fluorescent area beginning at 48 hours post-culture
initiation, rapidly reaching a plateau which was broadly
maintained for the remaining experimental time course. The
peaks and slow decreases in the fluorescent signal reflect the
replenishment of the calcein dye during media changes
followed by a small amount of bleaching due to the
fluorescent imaging. Differentiation as indicated by calcein
fluorescence could also be easily quantified for cells in rapid
osteogenic medium, with a significant rise following the
change to rapid osteogenic medium, reaching a plateau that
was maintained for the remainder of the experiment, with less
variation arising from media replenishment.
Figure 2: Expression of MSC and Osteogenic Differentiation Markers During Culture With Osteogenic Differentiation
Medium as Evaluated Using HTS by Cytometry. (A) Expression of markers of MSC identity when MSCs are cultured in rapid
osteogenic medium. (B) Quantification of alkaline phosphatase (ALPL) expression as an indicator of osteogenic
differentiation.
A
0d 3d 9d
B
0 2 4 6 8 10 1 2 14 16
0
5
10
15
Time (days)
Lipid dye (Total Area) x105
Adipogenic
Basal medium
7
Osteogenic differentiation can also be confirmed and
quantified by investigating cellular identity using cell surface
marker expression. MSC identity, representing a multipotent,
self-renewing population, is typically defined as positive
expression of CD90, CD73, and CD105, and lack of
expression of CD45 and CD34. Figure 2 shows the changes
in cellular marker expression during the differentiation period
using rapid osteogenic media. The decreases in positive
markers and increase in negative markers indicate a
reduction in the self-renewing MSC population as the
differentiation progressed.
ALPL is expressed at a low level in undifferentiated MSCs.
However, during the experiment there was a clear increase of
ALPL in the cells cultured in osteogenic medium and rapid
osteogenic medium compared to basal medium, indicating
that the cells were directed to the desired osteoblastic
cellular identity (Figure 2B). In rapid osteogenic medium,
ALPL expression was markedly increased above the control,
rising from around 25% positive at day 2 to around 70% at
day 17, indicating a substantial population of maturing
osteoblasts. ALPL is primarily expressed by osteoblasts
during the early stages of osteogenic differentiation and
bone formation. It is a marker of osteoblast activity and is
involved in the mineralization process. The reduction in ALPL
towards the end of the experiment with osteogenic medium
may be indicative of the osteoblast maturation into
osteocytes, which arise once osteoblasts are surrounded by
their own matrix. Once mature osteocytes become
embedded in the bone matrix, the expression of ALPL
significantly decreases.⁶
Real-Time Quantification of Adipogenic Differentiation
Adipogenic differentiation, similarly to osteogenic, is usually
detected using a destructive, endpoint histological stain
such as Oil Red O. These methods are semi-quantitative,
have low sensitivity for early differentiation, and are labor
intensive. A live-cell analysis approach is a desirable
alternative with obvious benefits. Here, a fluorescent lipid
dye was employed for the early detection of adipogenesis
through labeling of the characteristic formation of lipid
droplets within the cells.
Figure 3: Fluorescent Evaluation of Adipogenic Differentiation of MSCs Cultured in Adipogenic Differentiation Medium
Using Live-Cell Analysis. (A) Representative fluorescence and phase-contrast images of MSCs cultured either in adipogenic
medium or basal medium with fluorescent lipid dye, acquired at intervals during a 15-day period. (B) Quantification of
fluorescent lipid signal from images acquired during the differentiation period.
Figure 4: Analysis of Morphological Changes Accompanying Adipogenic Differentiation of MSCs Cultured in Adipogenic
Differentiation Medium Using Live-Cell Analysis. (A) Zoomed phase-contrast images of cells during the adipogenic
differentiation process. (B) Representative phase-contrast images of cells with overlaid AI Cell Segmentation mask (yellow
outlines). (C) Average phase object area at different time points. (D) Average object eccentricity at different time points.
A B C
0 3 7 10 13
0.75
0.80
0.85
0.90
Time (days)
Average Object Eccentricity
0 3 7 10 13
0
1000
2000
3000
4000
Time (days)
Avg Phase Object Area (µm²) D
0d 0h 0m
6d 0h 0m
10d 0h 0m
0d 0h 0m
6d 0h 0m
10d 0h 0m
8
The formation of lipid droplets within the differentiating
MSCs is distinctly visible in the images in Figure 3A. The
green fluorescent signal was detectable by day 3 following
culture initiation in the cells cultured with adipogenic
medium, with very low staining in the control basal medium
MSCs. Adipocyte morphology with internal lipid droplets can
be observed in the phase images, co-localized to the droplet
stains. The quantification of the lipid signal is presented in
Figure 5B. For the duration of the experiment lipid staining
in basal medium was negligible, in marked contrast to the
adipogenic media samples, which showed a larger total
fluorescent area from day 3 following the start of the
differentiation process. The peaks and slow decreases in
fluorescent staining here correspond to the replenishment
and bleaching of the stain, similar to the calcein.
Figure 5: Expression of MSC and Adipogenic Differentiation Markers During Culture With Adipogenic Medium as
Evaluated Using HTS by Cytometry. (A) Expression of markers of MSC identity. (B) Quantification of fluorescent lipid dye
and FABP4 as indicators of adipogenic differentiation.
A
Percentage positive (%)
CD90 CD73 CD105 CD45 CD34
0
20
40
60
80
100
120
Marker
P
erc
entag
e
p
ositiv
e (%)
Day 4
Day 6
Day 9
Day 11
Day 17
Day 2
120
100
80
60
40
20
0
CD90 CD73 CD105 CD45 CD34
Day 2
Day 4
Day 6
Day 9
Day 11
Day 17
Marker
B
Percentage positive (%)
2 4 6 9 11 17
0
20
40
60
80
100
Lipid
Time (days)
P
ercentag
e
p
ositive (%)
Adipogenic
Basal medium
100
80
60
40
20
0
2 4 6 9 11 14
Time (days)
Lipid
Percentage positive (%)
2 4 6 9 11 17
0
20
40
60
80
100
FABP4
Time (days)
P
ercentag
e
p
ositive (%)
Adipogenic
Basal medium
100
80
60
40
20
0
2 4 6 9 11 14
Time (days)
FABP4
9
In addition to employing fluorescent labeling of lipid droplets
to quantify adipocyte presence, their distinct morphology
Figure 4A allows a label-free approach to quantifying
adipogenesis. Figure 4 depicts how AI-based cell
segmentation can be used to evaluate key cell metrics such
as area and eccentricity on a population level and is able to
accurately segment low-contrast cells with heterogenous
morphologies until confluency is reached (Figure 4B).
As adipogenic differentiation progresses, cell morphology
changes from flattened, spindle-shaped cells to a greater
proportion of the cell population having a rounded or oval
shape. This is reflected in Figure 4C and D, with both area
and eccentricity initially high and decreasing as the time
course progresses. These two metrics, in combination with
visual assessment of the images, can be used to confirm
adipogenic differentiation without requiring the addition of
fluorescent reagents.
When examining expression of markers during adipogenic
differentiation, a distinct change in cellular identity was
observed during the process (Figure 5). As in the osteogenic
differentiation, expression of positive MSC markers remained
high for the duration of the experiment. However, both
negative markers, CD34 and CD45, were elevated from
4 days and higher levels were maintained until the adipocytes
became more mature.
The intracellular lipid dye can be detected using single cell
cytometry in addition to fluorescence imaging. By day 4 of
the process, 50% of the cells contained the dye, compared
to 0% in the basal medium control, which is a clear early
confirmation of adipogenesis. Concurrently, FABP4
expression was elevated in the adipogenic medium from
day 4 of the differentiation process.
Specifications subject to change without notice.
© Sartorius Lab Instruments GmbH & Co. KG.
Status: 09 | 2024
North America
Sartorius Corporation
565 Johnson Avenue
Bohemia, NY 11716
USA
Phone +1 734 769 1600
Email: orders.US07@sartorius.com
Europe
Sartorius UK Ltd
Longmead Business Centre
Blenheim Road
Epsom
Surrey, KT19 9QQ
United Kingdom
Phone +44 1763 227400
Email: euordersUK03@sartorius.com
Asia Pacific
Sartorius Japan K.K.
4th Floor, Daiwa Shinagawa North Bldg.
1-8-11, Kita-Shinagawa 1-chome
Shingawa-Ku
Tokyo 140-0001
Japan
Phone +813 6478 5202
Email: euordersUS07@sartorius.com
Find out more: sartorius.com/incucyte
sartorius.com/hts
For questions, email: AskAScientist@sartorius.com
Summary and Outlook
The use of advanced analysis technologies has significantly
enhanced the ability to assess MSC differentiation in
real-time, providing valuable insights into the dynamics
of the differentiation process. The successful application
of these technologies in both osteogenic and adipogenic
differentiation demonstrates their versatility and
effectiveness. By providing a comprehensive view of the
cellular changes, these methods pave the way for more
standardized, reliable, and reproducible assessments of MSC
functionality. These methods offer several advantages over
traditional endpoint assays, including quicker confirmation
of differentiation potential, preservation of cellular material
for further analysis, and the ability to monitor multiple
parameters simultaneously.
By adopting these advanced technologies, researchers
can improve the efficiency and depth of MSC research,
ultimately enhancing the therapeutic potential of MSCs
for treating various diseases and conditions.
References
1. Lee, D.H., Kim, S.J and Kim, S.A. et al. Past, present, and
future of cartilage restoration: from localized defect to
arthritis. Knee Surgery & Related Research. 2022.
Vol. 34, 1.
2. Kurtzberg J, Prockop S, Teira P, Bittencourt H, Lewis V,
Chan KW, Horn B, Yu L, Talano JA, Nemecek E, Mills CR,
Chaudhury S. Allogeneic human mesenchymal stem cell
therapy (remestemcel-L, Prochymal) as a rescue agent for
severe refractory acute graft-versus-host disease in
pediatric patients. Biol Blood Marrow Transplant. 2014.
Vol. Feb, 20(2). 229-35.
3. Yoshihisa Yamada, Shingo Minatoguchi, Hiromitsu
Kanamori, Atsushi Mikami, Hiroyuki Okura, Mari Dezawa,
Shinya Minatoguchi. Stem cell therapy for acute
myocardial infarction – focusing on the comparison
between Muse cells and mesenchymal stem cells.
Journal of Cardiology. 2022. Vol. 80, 1. 80-87.
4. Dominici, M., et al. Minimal criteria for defining
multipotent mesenchymal stromal cells. The International
Society for Cellular Therapy position statement.
Cytotherapy. 2006. Vol. 8, 4. 315-317.
5. White, Kristopher, et al. Calcein Binding to Assess
Mineralization in Hydrogel Microspheres. Polymers.
Basel: s.n., 2021. Vol. Jul 11, 13(14). 2274.
6. Franz-Odendaal, Tamara A, Hall, Brian K and Witten,
P Eckhard. Buried alive: How osteoblasts become
osteocytes. Developmental Dynamics. 2006.
Vol. Jan 235, 1. 176-90.
Brought to you by
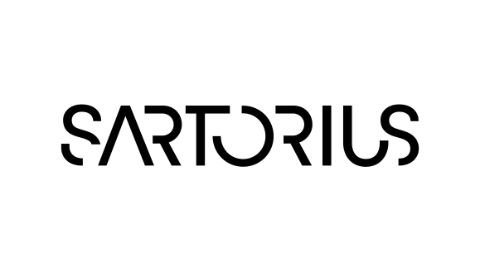
Download the Application Note for FREE Now!
*This also covers the processing of my personal data provided above for these purposes, including profile building to better tailor the communication to my personal interests based on my previous and predicted choices. Learn More
You can withdraw your consent at any time with effect for the future. This does not affect the lawfulness of the processing up to the time of withdrawal.
Further information on the processing of personal data can be found in the Sartorius Privacy Notice.
Technology Networks may contact you to offer you content or products based on your interest in this topic. You may opt-out at any time.
You can withdraw your consent at any time with effect for the future. This does not affect the lawfulness of the processing up to the time of withdrawal.
Further information on the processing of personal data can be found in the Sartorius Privacy Notice.
Technology Networks may contact you to offer you content or products based on your interest in this topic. You may opt-out at any time.
Experiencing issues viewing the form? Click here to access an alternate version